Love Me Love My Phages -Bacteriophages - The New Antibiotics-Part1
Love Your Phages
What Are Phages?
Phages (short form of Bacteriophages) are viruses that destroy many of the bacteria that create terrible harm in humans, animals and many of our wonderful plant species, and even our marvelous coral reefs. With the threat of global warming, there is also the fear that many of these bacteria borne diseases may increase their levels of fatal or disability creating effects.
History (From Wikipedia)
Since ancient times, there have been documented reports of river water having the ability to cure infectious diseases, such as leprosy. In 1896, Ernest Hanbury Hankin reported that something in the waters of the Ganges and Jumna rivers in India had marked antibacterial action against cholera and could pass through a very fine porcelain filter. In 1915, British bacteriologist Frederick Twort, superintendent of the Brown Institution of London, discovered a small agent that infected and killed bacteria. He considered the agent either 1) a stage in the life cycle of the bacteria, 2) an enzyme produced by the bacteria themselves or 3) a virus that grew on and destroyed the bacteria. Twort's work was interrupted by the onset of World War I and shortage of funding. Independently, French-Canadian microbiologist Félix d'Hérelle, working at the Pasteur Institute in Paris , announced on September 3, 1917 that he had discovered "an invisible, antagonistic microbe of the dysentery bacillus".
For d’Hérelle, there was no question as to the nature of his discovery: "In a flash I had understood: what caused my clear spots was in fact an invisible microbe ... a virus parasitic on bacteria." D'Hérelle called the virus a bacteriophage or bacteria-eater (from the Greek phagein meaning to eat). He also recorded a dramatic account of a man suffering from dysentery who was restored to good health by the bacteriophages. In 1926 in the Pulitzer-prize winning novel Arrowsmith, Sinclair Lewis fictionalized the application of bacteriophages as a therapeutic agent. Also in the 1920s the Eliava Institute was opened in Tbilisi , Georgia to research this new science and put it into practice. In 2006 the UK Ministry of Defence took responsibility for a G8-funded Global Partnership Priority Eliava Project as a retrospective study to explore the potential of bacteriophages for the 21st century. " (End of Wikipedia material)
The History of the Eliava Institute:
Eliava Institute itself is a fascinating story that I will leave for another article, but briefly The Institute was opened in Tbilisi , Georgia in 1923, and was a bacteriology laboratory. Its founder, Prof. George Eliava, was not aware of bacteriophages until 1926. In that year he met Felix d'Herelle during a visit to the Pasteur Institute in Paris . There, Eliava was enthusiastic about the potential of phage in the curing of bacterial disease, and invited d'Herelle to visit his laboratory in Georgia . Sadly Professor Eliava was executed by the Stalin Regime, but his works lives on today and bacteriophage samples are supplied to many countries, including Australia .
Phage Therapy
(From Wikipedia) "Phages were discovered to be anti-bacterial agents and put to use as such soon after they were discovered, with varying success. However, antibiotics were discovered some years later and marketed widely, popular because of their broad spectrum; also easier to manufacture in bulk, store and prescribe. Hence development of phage therapy was largely abandoned in the West, but continued throughout 1940s in the former Soviet Union for treating bacterial infections, with widespread use including the soldiers in the Red Army - much of the literature being in Russian or Georgian, and unavailable for many years in the West. This has continued after the war, with widespread use continuing in Georgia and elsewhere in Eastern Europe . There is anecdotal evidence there, but no completed clinical trials in the US or Western Europe ."
"Phage therapy has been attempted for the treatment of a variety of bacterial infections including: laryngitis, skin infections, dysentery, conjunctivitis, periodontitis, gingivitis, sinusitis, urinary tract infections and intestinal infections, burns, boils, etc.[2] - also poly-microbial biofilms on chronic wounds, ulcers and infected surgical sites." (End of Wikipedia)
Where Do We Go From Here?
Hopefully, a very long way. As the effectiveness of current antibiotics are rapidly reduced, death rates will rise. (In Australian Hospitals, the death rate from "Golden Staph" are around 20% and rising. There is a form of virulent TB that has a death rate of 85%, which is frightening, but both are treatable with Phage Therapy. Exciting research continues.
In Georgia, Poland, Russia, Israel, Australia , UK . France and other countries in Europe and the United States , just to name a few.
In Georgia, Poland, Russia, Israel, Australia
Gradually, I will pass on what I am learning, and we can all make sure that our Doctors, Nurses and other Health Specialists and Ministers of Health, and Hospitals will react quickly and save hundreds of thousands of lives.
Sadly, the 1,200 deaths from Cholera, recently in ZIMBABWE , were largely preventable., as it has been known for a very long time that Phage Therapy is very successful with this disease..
This Blog Site is dedicated to those who lost their lives in that epidemic and as a reminder that we must act as soon as we can, to especially insure that those suffering illnesses than can be prevented or treated by Phage Therapy, get this as soon as possible. And above all, in the Developing World, where antibiotics are often too expensive.
............................................................
Love Me Love My Phages
Love Me Love My Phages
..............................................................
In the 1920s and '30s, with diseases like dysentery and cholera running rampant, the discovery of bacteriophages was hailed as a breakthrough. Bacteriophages are viruses found virtually everywhere—from soil to seawater to your intestines—that kill specific, infection-causing bacteria. In the United States, the drug company Eli Lilly marketed phages for abscesses and respiratory infections. (Sinclair Lewis' Pulitzer-winning Arrowsmith is about a doctor who uses phages to prevent a diphtheria epidemic.) But by the 1940s, American scientists stopped working with phages for treatment because they no longer had reason to. Penicillin, discovered by the Scottish bacteriologist Alexander Fleming in 1928, had become widely available thanks to synthetic production and zapped infections without the expertise needed for finicky phages.
But now the equation has changed. Many kinds of bacteria have become antibiotic-resistant—prompting a few Western scientists, and patients, to travel to former Soviet Georgia to give bacteriophages for treatment a try. Phages have been used in the former Soviet Union for decades because scientists there had less access to antibiotics than their American and European counterparts did. Phages were a cheap alternative, and in Soviet clinical trials, they repeatedly stopped infections. Now in a bid for medical tourists, Georgia has opened a center in its capital, Tbilisi, which offers outpatient phage treatment to foreigners. In connection with the Eliava phage research institute, which Stalin helped set up in Tbilisi in 1923, the treatment center offers personalized cures for a host of infections the United States says it can no longer do anything about.
In 2000, the Centers for Disease Control, along with other federal agencies, warned that the world might soon return to a "pre-antibiotic era." Two million people each year now get hospital-borne bacterial infections, 1.4 million of them resistant to antibiotics and 90,000 of them lethal. One example is sepsis, the infection that sickened* Joan Didion's daughter, as Didion relates in The Year of Magical Thinking. New antibiotics are being discovered. But it takes 10 years and at least $800 million to bring an antibiotic to market, according to the Infectious Diseases Society of America. The big advantage that phages offer over antibiotics is that bacterial resistance is less of a problem. Unlike antibiotics, new phage batches can quickly be whipped up to take the place of phages to which bacteria become resistant.
The word phage comes from the Greek "to eat." A phage contains genetic material that gets injected into a virus's host. Whereas "bad" viruses infect healthy cells, phages target specific bacteria that then explode. At Eliava, phages are produced as a liquid that can be drunk or injected intravenously, as pills, or as phage-containing patches for wounds. Though few published articles in Western journals report positive clinical trials—most of the recent long-term research on phages comes out of the Soviet Union—some Western scientists say that phages are safe and that they work. "There is no evidence that phage is harmful in any way," says Nick Mann, a biology professor at the University of Warwick in England and co-director of phage R&D company Novolytics.
So, why do American patients need to go to all the way to Georgia for treatment? For starters, in their natural state phages are hard to patent, the route by which drug companies lock up future profits. The first company to spend millions of dollars to prove that a particular phage is safe could allow its competitors to capitalize on the results. As important is the difficulty of regulation. There are two ways that phages are currently used in the former Soviet Union, and both pose problems from the point of view of the Food and Drug Administration. At the Tbilisi phage center, phages are personalized: You send your bacterial sample to the lab, and it's either matched up with an existing phage or a phage is cultured just for you. In the United States, by contrast, drugs are mass produced, which makes it easier for the FDA to regulate them.
Phages are also sold over-the-counter in Georgia. People take the popular mixture piobacteriophage, for example, to fight off common infections including staph and strep. These phage mixtures are updated regularly so they can attack newly emerging bacterial strains. In the United States, the FDA would want the phages in each new concoction to be gene sequenced, because regulations require every component of a drug to be identified. To do so would entail prohibitively expensive and lengthy clinical trials.
In the early years of phage research in the United States, says former National Institutes of Health scientist Carl Merril, bacteriophages allegedly killed more people than they cured. Phages are culled from dirty, wet places—the first was found in the Ganges River—a recipe for infection unless you know what you're doing. And some kinds of phages—called lysogenic phages—are potentially dangerous, because they sometimes carry genes that cause bacteria to release toxins. So, there is reason for caution.
Despite the caveats, a number of phage biotechnology firms have recently opened up in the United States and also in countries like Canada and Israel. Phage biologists point out we know much more about phage biology now than when the viruses were first discovered. Methods of using phages for treatment, from distillation to identification, have improved significantly since then. Clinicians worldwide also report that patients using phages have had good recovery rates and minor or fleeting side effects. Evergreen State College professor Elizabeth Kutter, who collaborates closely with Eliava researchers in Georgia and heads an international phage conference each year, is working with others to find ways to commercialize phages that could sidestep some of the problems with patenting and regulation. Flu vaccine offers one instructive possibility. Like over-the-counter phages, the vaccine is updated regularly with the most recent strain of flu virus—without requiring FDA approval each time.
There are already multiple uses for phages without FDA approval. A promising area is American agriculture and livestock, which is regulated by the less stringent United States Department of Agriculture. Domestic scientists are looking at ways in which phages could kill bacteria before they cause infection (rather than fight an infection after it has begun). Alexander Sulakvelidze, an assistant medical professor at the University of Maryland and a co-founder of the phage R&D company Intralytix, awaits federal approval for a phage-based wash for meat and produce that protects against food poisoning. Vincent Fischetti, a professor at the Rockefeller Institute, is designing a phage-based enzyme solution that can be sprayed into the noses and mouths of hospital and nursing-home patients. Fischetti and researchers in Tbilisi are also experimenting with using phages to detect anthrax and cholera in the case of a terrorist attack.
Using phages to treat infections at home, on the other hand, for the moment seems unlikely. One company recently tried to open a phage center in Tijuana but was deterred by the Mexican government. Phages might be offered someday at clinics on Native American reservations, as a casino-like quirk of legislative autonomy. But for now, U.S. patients at a loss for options may decide that Tbilisi is close enough.
Correction, June 1, 2006: The original sentence stated that Didion's daughter died of sepsis. In fact, she got sick from sepsis but died later from another condition. Click here to return to the corrected sentence.
Love Me Love My Phages
................................................................
Phage therapy is the therapeutic use of bacteriophages to treat pathogenic bacterial infections. Although extensively used and developed mainly in former Soviet Union countries for about 90 years, this method of therapy is still being tested elsewhere for treatment of a variety of bacterial and poly-microbial biofilm infections, and has not yet been approved in countries other than Georgia. Phage therapy has many potential applications in human medicine as well as dentistry, veterinary science, and agriculture.[1] If the target host of a phage therapy treatment is not an animal, however, then the term "biocontrol" (as in phage-mediated biocontrol of bacteria) is sometimes employed rather than "phage therapy".
An important theoretical benefit of phage therapy is that bacteriophages can be much more specific than more common drugs, so can be chosen to be harmless to not only the host organism (human, animal, or plant), but also other beneficial bacteria, such as gut flora, reducing the chances of opportunistic infections. They also have a high therapeutic index, that is, phage therapy gives rise to few if any side effects, as opposed to drugs, and does not stress the liver. Because phages replicate in vivo, a smaller effective dose can be used. On the other hand, this specificity is also a disadvantage: A phage will only kill a bacterium if it is a match to the specific strain. Thus, phage mixtures are often applied to improve the chances of success, or samples can be taken and an appropriate phage identified and grown.
Phages are currently being used therapeutically to treat bacterial infections that do not respond to conventional antibiotics, particularly in the country of Georgia.[2][3][4] They tend to be more successful than antibiotics where there is a biofilm covered by a polysaccharide layer, which antibiotics typically cannot penetrate.[citation needed] In the West, no therapies are currently authorized for use on humans, although phages for killing food poisoning bacteria (Listeria) are now in use.[5]
In neighbouring countries including Russia, extensive research and development soon began in this field. In the USA during the 1940s, commercialization of phage therapy was undertaken by the large pharmaceutical company, Eli Lilly.
Whilst knowledge was being accumulated regarding the biology of phages and how to use phage cocktails correctly, early uses of phage therapy were often unreliable. When antibiotics were discovered in 1941 and marketed widely in the USA and Europe, Western scientists mostly lost interest in further use and study of phage therapy for some time.[7]
Isolated from Western advances in antibiotic production in the 1940s, Russian scientists continued to develop already successful phage therapy to treat the wounds of soldiers in field hospitals. During World War II, the Soviet Union used bacteriophages to treat many soldiers infected with various bacterial diseases e.g. dysentery and gangrene. The success rate was as good as, if not better than any antibiotic.[citation needed] Russian researchers continued to develop and to refine their treatments and to publish their research and results. However, due to the scientific barriers of the Cold War, this knowledge was not translated and did not proliferate across the world.[8][9]
There is an extensive library and research center at the Eliava Institute in Tbilisi, Georgia. Phage therapy is today a widespread form of treatment in neighbouring countries. For 80 years Georgian doctors have been treating local people, including babies and newborns, with phages.
As a result of the development of antibiotic resistance since the 1950s and an advancement of scientific knowledge, there is renewed interest worldwide in the ability of phage therapy to eradicate bacterial infections and chronic polymicrobial biofilm, along with other strategies.
Phages have been explored as means to eliminate pathogens like Campylobacter in raw food[10] and Listeria in fresh food or to reduce food spoilage bacteria.[11] In agricultural practice phages were used to fight pathogens like Campylobacter, Escherichia and Salmonella in farm animals, Lactococcus and Vibrio pathogens in fish from aquaculture and Erwinia and Xanthomonas in plants of agricultural importance. The oldest use was, however, in human medicine. Phages were used against diarrheal diseases caused by E. coli, Shigella or Vibrio and against wound infections caused by facultative pathogens of the skin like staphylococci and streptococci. Recently the phage therapy approach has been applied to systemic and even intracellular infections and the addition of non-replicating phage and isolated phage enzymes like lysins to the antimicrobial arsenal. However, definitive proof for the efficiency of these phage approaches in the field or the hospital is only provided in a few cases.[11]
Some of the interest in the West can be traced back to 1994, when Soothill demonstrated (in an animal model) that the use of phages could improve the success of skin grafts by reducing the underlying Pseudomonas aeruginosa infection.[12] Recent studies have provided additional support for these findings.[13]
Recently, the use of phages as delivery mechanisms for traditional antibiotics has been proposed.[14][15] The use of phages to deliver antitumor agents has also been described, in preliminary in vitro experiments for cells in tissue culture.[16]
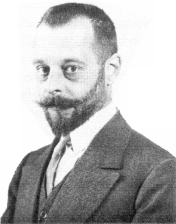
In addition, due to the specificity of individual phages, for a high chance of success, a mixture of phages is often applied. This means that 'banks' containing many different phages are needed to be kept and regularly updated with new phages, which makes regulatory testing for safety harder and more expensive.
Illustrations below are Frederick Twort and Felix d'Hérelle
Love Me Love My Phages
...........................................................................................
Before the development of modern antimicrobials, phage therapy was considered a feasible option for the treatment of bacterial infection. The study team will therefore use their improved understanding of the interactions among hosts, V. cholerae,and cholera phages to identify new ways of preventing cholera epidemics
Phage in the Time of Cholera
Joshua S. Weitz
, and Hyman Hartman
Department of Ecology and Evolutionary Biology,
Princeton University, Princeton, NJ 08544.
Email: jsweitz@princeton.edu
*
Corresponding author
2
Center for Biomedical Engineering,
Massachusetts Institute of Technology, Cambridge, MA 02139.
Email: hhartman@mit.edu
January 27, 2006
Bacteriophage (bacterial viruses) were heralded as revolutionary therapeutic agents soon
after the discovery by Félix d’Herelle in 1917 of an “invisible microbe”
capable of lysing bacteria
1
. Bacteriophage appeared to be efficient killers of their bacterial hosts –
we now know that their life history is far more complex than first
assumed
2
– and so the effort to use phage as curatives or prophylaxis spread
quickly to research institutes in Europe, North America, and Asia
3
. d’Herelle
himself spearheaded many of these efforts, the most famous of which was the
initiation of an extensive campaign to use phage in the treatment and prevention of
cholera in colonial India. The authors of one such study conclude by noting that
“the results establish sufficient probability in favour of a significant effect of the
administration of bacteriophage to form a basis of practical policy in the treatment
and prevention of cholera in villages”
4
. The early hopes never fulfilled
expectations, for both clinical and political reasons
3
, and the eventual development
of broad spectrum antibiotics provided a more reliable, effective means of
controlling bacterial infections. The rise of antibiotic resistance has, in turn,
revived interest in bacteriophage therapy despite concerns and uncertainties as to
its effectiveness
5
. We consider here an alternative approach to modern
bacteriophage therapy, by revisiting the idea of inoculating bacteriophage directly
into the environment.
Most tests, theories, and proposals to implement bacteriophage therapy
regard the human body as the potential site for intervention
6,7
. But for many
bacterial diseases affecting human health, the pool of infecting bacteria comes
from water, soils, food, and other host organisms; some of these potential sources
of infection do not possess a complex immune system capable of selectively
eliminating foreign agents. In contrast to agricultural settings where environmental
application of phage as biocontrol is already being considered
8
, we believe there
exists an as yet overlooked opportunity to reduce the severity, extent, and
persistence of some bacterial epidemics by developing ecological-based cures for
human disease.
A suitable target disease is cholera. Recent studies have demonstrated a
significant correlation between the increase in density of cholera-specific phage
and the decrease in density of Vibrio cholerae (in both water sources and fecal
matter from infected patients)
9,10
. The reasons are apparently simple: presence of
V. cholerae provides an opportunity for the spread and increase of phage which
leads to decreasing host density, which in turns leads to the washout/death of
phage . A comprehensive description of cholera disease dynamics involves many
factors including environmental seasonality
11
, long-distance dispersal mediated by
alternative hosts
12
, as well as life-history modalities that enable V. cholerae to
respond to stressful conditions
13
. Without diminishing the importance of these and
other factors, in the case of cholera it is apparent that phage and bacteria go
through alternating boom-and-bust cycles. What are the practical steps of
intervention so as to minimize the likelihood of devastating epidemic booms of V.
cholerae ?
Briefly, the peak of phage lags behind the peak of bacteria. Growing up O1
and/or O139 serogroup-specific phage in the lab, therapy by the flask as it were,
and then adding phage to at-risk water sources may augment the ability of phage to
keep pace with the dynamics of its host and suppress the spread of an epidemic. In
a sense, we are suggesting altering the “natural course”
10
of host-phage population
dynamics with an artificial injection of phage. The utility and effectiveness of any
such ecological inoculation depend on careful balancing of environmental
connectivity of infected areas, risks to human populations, as well as the life-
history and parameterization of the biocontrol agent themselves. Ultimately,
limiting and/or eliminating an undesirable bacterial population constitutes a
problem in coevolutionary biological control. Recent theoretical work on
coevolutionary dynamics of bacteria and bacteriophage in simple aquatic
environments demonstrates that coevolution-induced outcomes, e.g. eradication of
phage and host, sequential strain replacement, or host-phage diversification,
depend on characterizing (and possibly manipulating) rates of mutagenesis, host
growth rate and strain-specific adsorption rates, and host-range characteristics of
mutants
14
. However, the ecology of natural environments is far more complex.
Likely sites for intervention include sources of drinking water, wells, and sewage
systems so as to minimize the flow of bacterial agents into water used for drinking
and bathing. Assessments of the lifetime of phage in local habitats would be
necessary as conditions (e.g., temperature, salinity, pH) change over the course of
intervention. In addition, the ecohydrology of the affected region may be
important, as intervention strategies will depend on whether disease outbreaks are
localized to isolated sites, linked to seasonal flooding, or occur along riverine
corridors.
These concerns notwithstanding, cholera-specific phage are already found
in natural environments and there exists strong evidence to suggest that their
presence leads to the decline of cholera epidemics
10
. The risks associated with
ecological bacteriophage therapy should be mitigated by the use of virulent, in
contrast to temperate, strains of phage. In this regard, the previously identified lytic
phages JSF1 and/or JSF5 specific to V. cholerae serogroup O1 seem ideal
candidates for initial studies
10
. If the origins of seasonal cholera epidemics are
harbored within environmental pools, then efforts should be made to seek out the
most effective means of adding bacteriophage to eliminate the incubation and
growth of V. cholerae populations when they are at their most vulnerable.
Diminishing the density of V. cholerae would also be important to impeding the
spread of disease, since the infectious dose is generally considered to be on the
order of 10
4
bacterial cells. Thus far, the spread of cholera has been mitigated by
improvements in water quality, low-cost preventative measures in at-risk regions,
e.g., filtering water through sari cloth
15
, as well as by improvements in post-
infection treatment, e.g., single-dose antibiotic therapy
16
, though the global cholera
pandemic has not abated. Bacteriophage could become an additional tool in the
public health struggle against cholera. The initiation of controlled experiments that
incorporate recent advances in the genetics and evolutionary ecology of phage may
offer hope that d’Herelle’s early mission to eradicate cholera in the Indian
subcontinent need not have been in vain.
References
[1] d’Herelle, F. Sur un microbe invisible antagoniste des bacilles dysentèriques.
Cr. R. Acad. Sci. Paris 165 (1917).
[2] Weinbauer, M. Ecology of prokaryotic viruses. FEMS Microbiology Reviews
28, 127–81 (2004).
[3] Summers, W. Félix d’Herelle and the Origins of Molecular Biology (Yale
University Press, 1999).
[4] Morison, J., Rice, E. & Pal Choudhury, B. Bacteriophage in the treatment and
prevention of cholera. Indian Journal of Medical Research 21, 790–907 (1934).
[5] Thiel, K. Old dogma, new tricks – 21st Century phage therapy. Nature
Biotechnology 22, 31–6 (2004).
[6] Merril, C., Scholl, D. & Adhya, S. The prospect for bacteriophage therapy in
Western medicine. Nature Reviews Drug Discovery 6, 489– 97 (2003).
[7] Levin, B. & Bull, J. Population and evolutionary dynamics of phage therapy.
Nature Reviews Microbiology 2, 166–73 (2004).
[8] Goodridge, L. & Abedon, S. Bacteriophage biocontrol and bioprocessing:
application of phage therapy to industry. SIM News 53, 254–62 (2003).
[9] Faruque, S. et al. Seasonal epidemics of cholera inversely correlate with the
prevalence of environmental cholera phages. Proceedings of the National Academy
of Sciences, USA 102, 1702–7 (2005).
[10] Faruque, S. et al. Self-limiting nature of aseasonal cholera epidemics: Role of
host-mediated amplification of phage. Proceedings of the National Academy of
Sciences, USA 102, 6119–24 (2005).
[11] Koelle, K., Rodó, Pascual, M., Yunus, M. & Mostafa, G. Refractory periods
and climate forcing in cholera dynamics. Nature 436, 696–700 (2005).
[12] Colwell, R. Global climate and infectious disease: the cholera paradigm.
Science 274, 2025–31 (1996).
[13] Roszak, D. & Colwell, R. Survival strategies of bacteria in the natural
environment. Microbiology Reviews 51, 365–79 (1987).
[14] Weitz, J., Hartman, H. & Levin, S. Coevolutionary arms races between
bacteria and bacteriophage. Proceedings of the National Academy of Sciences,
USA 102, 9535–40 (2005).
[15] Colwell, R. et al. Reduction of cholera in Bangladeshi villages by simple
filtration. Proceedings of the National Academy of Sciences, USA 100, 1051–1055
(2003).
[16] Saha, D. et al. Single-dose ciproflaxacin versus 12-dose erythromycin for
childhood cholera: a randomised controlled trial. The Lancet DOI:10.1016/S0140–
6736(05)67290–X (2005).
Love Me Love My Phages
.........................................................................................
Sunday, November 26, 2000
The Polish investigators have been rigorous in matching the phages to the bacterial strain infecting the given patients. Their practice, as stated in the published reports, is to culture the bacteria during the course of treatment, so that the occurrence of a mutant resisting the phage can be countered by switching to a different phage strain. The group also has panels of multivalent
phages available, for use in fulminant infections (such as septicemia with acute respiratory distress syndrome) where time is insufficient to classify the offending bacteria or to match phages to bacteria.
The group now has statistics on the treatment of approximately 1 300 cases. The overall cure rate across the spectrum of pathogens and sites of infection is approximately 86% (personal communication from Dr. B. Weber-Dabrowska). A criticism of the work by Slopek’s group is that - the absence of placebo controls means the power of suggestion cannot be definitively ruled-out. It is clear that the difficulties of that nation’s economy over recent decades has denied the investigators the financial resources needed to enroll matched cohorts in a placebo arm of a clinical trial. While the criticism is valid, and absolute proof of principle can be obtained only through placebo-controlled trials, nevertheless the usefulness of the data is improved by the detailed statistical accounting of the percentages of complete, partial and nil response. One of the factors that enables this author to find the data from Poland more believable (even in the absence of double-blind proof) is that in conditions such as emphysema where phage efficacy might be somewhat impeded, the group’s statistics show that the success rate is considerably lower than for other conditions where such impediments do not obtain*.
The Republic of Georgia.
The work started in Tblisi in the 1930s by d’Hérelle and his Georgian colleague, Eliava, continues to this day. In the 1970s, under the direction of Dr. Teimuraz Chanishvili, the Eliava-d’Hérelle Institute had a large staff manufacturing considerable quantities of phage preparations per year, primarily for the control of dysentery in the troops of the Soviet Army. This group has anecdotal evidence of the efficacy of phage therapy. They report, for example, that in certain adult and pediatric hospitals it is routine for their phage preparations to be administered topically on surgical incisions. Given the lack of statistical analysis, there is little to be said other than the anecdotal reports are encouraging that phage therapy can be useful.
Another example is vancomycin intermediate-resistant Staphylococcus aureus (VISA), strains of which have recently emerged in three nations (Japan, U.S. and Scotland), and are known to have killed 4 patients to date. Such strains spread throughout Japanese hospitals within a year of their first appearance.
Unfortunately, it has been demonstrated that some hospital strains of methicillin-resistant S. aureus (MRSA) that are widespread have become vancomycin resistant upon exposure of the patients to vancomycin 1, 2.
Experts predict that S. aureus will progress to become completely resistant to vancomycin (the antibiotic of last resort for most strains of this pathogen), and that when this occurs, millions of people will die each year from infections that had until recently been fairly easy to control. Based on such developments and impending developments with pathogens such as MRSA and VRE, opinion leaders have been warning that we are entering the “Post-Antibiotic Era”.
While pharmaceutical companies are developing new antibiotics to counter the trend, it has been shown that half a century of global antibiotic abuse has equipped the surviving bacteria with “supergenes” that enable them to quickly resist new classes of antibiotics, even those to which they have never been exposed 1.
Examples of the “supergenes” are mutations that 1) enable bacteria to pump out several classes of antibiotics (through an efficient efflux pump), or that 2) alter the antibiotic binding sites on ribosomal subunits, so that several different classes of antibiotics can no longer inhibit those subunits. As a consequence, in recent years, by the time newer antibiotics have gone through
clinical trials and have reached the market, 20% or more of clinical isolates in the hospitals are already resistant to them at the time of regulatory approval, and within a few more years the majority of strains are resistant.
Future Prospects for Phage Therapy
Infectious disease experts have warned that there is now a compelling need to develop totally new classes of antibacterial agents, ones that cannot be resisted by the same genes that render bacteria resistant to antibiotics.
Phage therapy represents such a “new” class. We believe that the impediments cited above (bacterial debris in the preparations, rapid clearance in the body, etc.) can be overcome, freeing up the phages so that their attributes (such as exponential growth, and the ability to mutate against resistant bacteria) can be used to great advantage.
There are 3 additional attributes of phages that should be noted: Host specificity. While the host specificity is somewhat of a drawback (requiring a match up of phage to bacterial target, and/or the development of highly multi-valent phages), it also offers the great advantage that the phages will not kill other species of bacteria.
* Conditions where phage efficacy is predicted to be reduced would include 1) hypoxic sites, where bacterial replication is slower and therefore phage replication is reduced; and 2) chronic obstructive pulmonary disease, where high acidity and proteases would be expected to inactivate some percentage of the phages.
Thus, e.g., phage therapy is not likely to kill off the healthy flora of the intestines, lungs or urogenital tract, and it is therefore unlikely to provoke the illnesses and deaths seen when antibiotics cause overgrowth of pathogens (such as Clostridia difficile and Candida albicans).
Genetic engineering. It is possible to genetically engineer phages to express new traits of potential value. In so doing, scientists will have to deal with the legitimate concerns of regulatory agencies concerning recombinant organisms. The regulatory obstacles may be well worth the price, given the powerful engineering tools that are currently available.
Ideal candidates for co-therapy with antibiotics. If a given bacterium acquires resistance to a phage (e.g. by a mutation in the receptor site or in the endonuclease enzymes), that mutation is not likely to “teach” the bacterium to resist the antibiotics (which do not target those structures). Similarly, if a given bacterium acquires resistance to an antibiotic (e. g. by a mutation in the reflux pump or in the ribosomal subunits), that mutation is not likely to “teach” the bacterium to resist the phage (which does not target those structures). Thus, if the bacterium is exposed to both agents, the odds are remote that any resistance genes it starts to express (or acquires anew) will enable it to survive. There are reports that bacteria tend to mutate against antibiotics once in every 106 divisions, while they tend to mutate against phages once in every 107 divisions.
Therefore the odds of a given bacterium mutating against a phage and an antibiotic at the same time would be the product of 106×107, meaning it would likely take 1013 bacterial divisions for such a double mutation to occur. Given that low probability, the co-administration of phages and antibiotics may help prevent the emergence of bacterial resistance to antibiotics, thereby greatly prolonging their clinical usefulness (and vice versa). Just as multiple classes of anti-HIV medications are administered to AIDS patients, to prevent the emergence of resistant strains of that virus, so it is that co-therapy with phages and antibiotics may also prove to be of great clinical value.
Conclusion
Multidrug-resistant bacteria have opened a second window for phage therapy. Modern innovations, combined with careful scientific methodology, can enhance mankind’s ability to make it work this time around. Phage therapy can then serve as a stand-alone therapy for infections that are fully resistant. It will also then be able to serve as a co-therapeutic agent for infections that are still susceptible to antibiotics, by helping to prevent the emergence of bacterial mutants against either agent.
References
1. ACKERMANN H. -W. and DUBOW M. (1987): Viruses of prokaryotes
I: General properties of bacteriophages (chapter 7). Practical
applications of bacteriophages. CRC Press, Boca Raton,
Florida.
2. ALISKY J. et al. (1998): Bacteriophages show promise as antimicrobial
agents. J. Infect., 36, 5–15.
3. BARROW P. A. and SOOTHILL J. S. (1997): Bacteriophage therapy
and prophylaxis: rediscovery and renewed assessment of
the potential. Trends Microbiol., 5, 268–271.
4. BERCHIERI A. et al. (1991): The activity in the chicken alimentary
tract of bacteriophages lytic for Salmonella typhimurium.
Res. Microbiol., 142, 541–549.
5. D’HÉRELLE F. (1917): Sur un microbe invisible antagoniste des
bac. dysentériques. Crit. Rev. Acad. Sci. Paris, 165, 373.
6. D’HÉRELLE F. (1922): The bacteriophage: its role in immunity.
Williams and Wilkens Co. /Waverly Press, Baltimore, USA.
7. GEIER M., FRIGG M. E. and MERRIL C. (1973): Fate of bacteriophage
lambda in non-immune germ-free mice. Nature, 246,
221–222.
8. LEDERBERG J. (1996): Commentary. Proc. Natl. Acad. Sci.
USA, 93, 3167–3168.
9. LEVIN B. and BULL J. J. (1996): Phage therapy revisited: the
population biology of a bacterial infection and its treatment
with bacteriophage and antibiotics. Am. Naturalist, 147, 881–898.
10. LEVY S. (1992): The antibiotic paradox. Plenum Press, New
York.
11. MERRIL C. et al. (1996): Long-circulating bacteriophage as
antibacterial agents. Proc. Natl. Acad. Sci. USA, 93, 3188–
2192.
12. MURRAY B. (1998): Diversity among multidrug-resistant Enterococci.
Emerging Infect. Dis., 4, 37–47.
13. SHRAYER D. (1996): Felix d’Hérelle in Russia. Bull. Inst. Pasteur,
94, 91–96.
14. SMITH H. W. and HUGGINS R. B. (1982): Successful treatment
of experimental E. coli infections in mice using phage: its general
superiority over antibiotics. J. Gen. Microbiol., 128, 307–318.
15. SMITH H. W. and HUGGINS R. B. (1983): Effectiveness of
phages in treating experimental E. coli diarrhoea in calves, piglets
and lambs. J. Gen. Microbiol., 129, 2659–2675.
16. SMITH H. W. and HUGGINS R. B. (1987): The control of experimental
E. coli diarrhea in calves by means of bacteriophage.
J. Gen. Microbiol., 133, 1111–1126.
17. SMITH T. et al. (1999): Emergence of vancomycin resistance in
Staphylococcus aureus. N. Engl. J. Med., 340, 493–501.
18. SOOTHILL J. S. (1992): Treatment of experimental infections of
mice with bacteriophages. Med. Microbiol., 37, 258–261.
19. SUMMERS W. C. (1998): D’Hérelle. Yale University Press (in
press).
20. S´LOPEK S. and KUCHAREWICZ-KRUKOWSKA A. (1987): Immunogenic
effect of bacteriophage in patients subjected to phage
therapy. Arch. Immunol. Ther. Exp., 35, 553–561.
21. S´LOPEK S., KUCHAREWICZ-KRUKOWSKA A., WEBER-DA˛BROWSKA
B. and DA˛BROWSKI M. (1985): Results of bacteriophage
treatment of suppurative bacterial infections. IV. Evaluation of
R. M. Carlton: Phage Therapy in the Past and Future 273
the results obtained in 370 cases. Arch. Immunol. Ther. Exp.,
33, 219–240.
22. S´LOPEK S., KUCHAREWICZ-KRUKOWSKA A., WEBER-DA˛BROWSKA
B. and DA˛BROWSKI M. (1985): Results of bacteriophage
treatment of suppurative bacterial infections VI. Analysis of
treatment of suppurative staphylococcal infections. Arch. Immunol.
Ther. Exp., 33, 261–273.
23. S´LOPEK S., WEBER-DA˛BROWSKA B., DA˛BROWSKI M. and KUCHAREWICZ-
KRUKOWSKA A. (1987): Results of bacteriophage
treatment of suppurative bacterial infections in the years 1981–
1986. Arch. Immunol. Ther. Exp., 35, 569–583.
24. VAN HELVOORT T. (1992): Bacteriological and physiological
research styles in the early controversy on the nature of the
bacteriophage phenomenon. Med. Hist., 36, 243–270.
25. WALDVOGEL F. (1999): New resistance in Staphylococcus
aureus. N. Engl. J. Med., 340, 556–557.
26. WEBER-DA˛BROWSKA B., DA˛BROWSKI M. and S´ LOPEK S.
(1987): Studies on bacteriophage penetration in patients subjected
to phage therapy. Arch. Immunol. Ther. Exp., 35, 563–568.
Received in May 1999
Accepted in June 1999
George Eliava Institute -Tbilisi, Georgia
(From Wikipedia, the free encyclopedia)
“The Tbilisi Institute, now called the George Eliava Institute of Bacteriophage, Microbiology and Virology (IBMV) has been active since the 1930s in the field of phage therapy, which is used to combat microbial infection (cf. antibiotic-resistant strains).”
History
“The Institute was opened in Tbilisi, Georgia in 1923, and was a bacteriology laboratory. Its founder, Prof. George Eliava, was not aware of bacteriophages until 1926. In that year he met Felix d'Herelle during a visit to the Pasteur Institute in Paris. There, Eliava was enthusiastic about the potential of phage in the curing of bacterial disease, and invited d'Herelle to visit his laboratory in Georgia.”
“D'Herelle visited Tbilisi twice in 1934-35, and agreed to work with Prof. Eliava. It has been suggested the d'Herelle became enamoured of the communist idea. In 1934, Stalin invited d'Herelle to the Institute in Tbilisi; he accepted and worked there part-time for about a year - and even dedicated one of his books, "The Bacteriophage and the Phenomenon of Recovery," written and published in Tbilisi in 1935, to Stalin.”
“D'Herelle had planned to take up permanent residence in Tbilisi and had started to build a cottage on the grounds of the Institute (it would later house the KGB's Georgian headquarters).”
“However, the collaboration between the two scientists was not to be. Around the time d'Herelle was to take up residence, George Eliava became involved romantically with the woman that the head of Stalin's secret police, Lavrenti Beria, was also in love with. Eliava's fate was decided. He was executed and denounced as a supposed enemy of the people. D'Herelle fled from Tbilisi and, some believe, never returned. Another account states that he was in Paris at the time of Eliava's execution, and decided not to return. D'Herelle's book was also banned from distribution.”
“In spite of this development, the Institute did not change its practical specialization, and continued its activity in the field of bacteriophage research. In 1938, the Institute of Bacteriophage Research and the Institute of Microbiology & Epidemiology (founded separately in 1936) merged, and the Institute of Microbiology, Epidemiology and Bacteriophage was formed. It existed until 1951 and was authorized by the People's Commissary of Health of Georgia. After 1951, it came under the auspices of the All-Union Ministry of Health and was renamed The Institute of Vaccine and Sera.”
“Since its inception, the Institute was composed of a combination of industrial and scientific (research) departments. In 1988 the Institute was rearranged again and emerged as the Scientific Industrial Union "Bacteriophage" (SIU "Bacteriophage"). Around that time, its scientific portion was renamed the George Eliava Research Institute of Bacteriophage.”
“Based on the original intentions of D'Herelle and Eliava, the Bacteriophage Institute retained its leadership among other institutes of similar profile over the years. Teimuraz Chanishvili was the leader of the scientific part of the Institute for over 30 years, until his death in August 2007. His niece, Nino Chanishvili has since taken over operations.”
The institute behind the Iron Curtain
“The Institute in Tbilisi became a general Soviet institute for the development and production of bacteriophage drugs. Patients with serious infectious diseases came from all over the Soviet Union to receive treatments there, which were reportedly successful. Bacteriophages became a routine part of treatment in clinics and hospitals. Ointments for the skin, and pills, drops, and rinses consisting of phages were sold and are still sold at pharmacies throughout Eastern Europe at incredibly low prices.” “These therapies are very effective, completely harmless to humans, and are much cheaper than antibiotics. Further, much of this therapy is apparently available without a doctor's prescription.”
“As the world is well aware, the Soviet Union fell apart within 2 years after the fall of the Berlin Wall in 1989. In 1991, after the Republic of Georgia declined to join the Russian Federation and civil war broke out there, the Tbilisi facility was essentially ruined. The Eliava Institute's facilities were damaged and decades of research on bacteriophage nearly went down the drain. Thousands of bacteriophage samples identified over the years and catalogued in huge, refrigerated "libraries" suffered irreversible damage due to frequent electrical outages. Apparently, the Russians transferred some of the equipment to their territory and built plants for the production of bacteriophages in other locations. Clearly, they recognized the importance of the research and also that of continued bacteriophage therapy. The situation at the Eliava Institute continued to deteriorate until it was on the verge of closure.”
“
However, in 1997, a report on the Institute was broadcast by the BBC, sparking a flurry of media interest in the West. The headlines drew doctors and scientists to Tbilisi - and also, most importantly, energetic entrepreneurs from around the world who were determined to help save the Institute and its stocks and fully explore the potential of this "new" and highly effective therapy. Georgian scientists whose names were connected in some way to the Institute saw great opportunity, and some of them emigrated to the West to be part of joint projects. Some of the Institute's projects with the rest of the world can be seen on the website of the Georgian Academy of Sciences, the umbrella entity which now includes the Eliava Institute. The URL is http://www.eliava-institute.org/
Address
“The George Eliava Institute is located at:
3, Gotua Street
380060, Tbilisi
Georgia
Tel: +995 32 37 42 27 or +995 32 23 32 95 Fax: +995 32 99 91 53 or +995 32 22 19 6
External links
A few of the companies who are or have been connected to the Eliava Institute and/or its phage research are as follows:
References
Nature Publishing Group: www.nature.com/naturebiotechnology. Volume 22, No. 1, Jan. 2004, Old Dogma, New Tricks - 21st Century Phage Therapy, by Karl Thiel.
“Proc. Natl. Acad. Sci. USA: Vol. 93, pp. 3167-3168, April 1996, Smaller fleas...ad infinitum: Therapeutic Bacteriophage Redux, by Joshua Lederberg, Raymond and Beverly Sackler Foundation Scholar at the Rockefeller University, 1230 York Avenue, New York, NY 10021.
The bactericidal action of the waters of the Jamuna and Ganga rivers on Cholera microbes, By M.E Hankin, Government Laboratory, Agra, India. Translated from the original article published in French, Ref. Ann. De l’ Inst. Pasteur 10.511 (1896).”
(END OF WIKIPEDIA MATERIAL)
...................
Phage Therapy
Phage Therapy involves carefully chose and tested species of Bacteriophages, which are matched to the specific bacteria, which is causing the infection, inflammation, swelling or producing toxins, and causing other ill-effects.
Phage Therapy (which will be covered in succeeding pages over time), like antibiotics, can only tackle infections due to bacteria and not viruses. But when infected by a virus infection, we may become prone to deadly bacterial infections, which may actually kill us, rather than the virus. Secondary infections treated by Phage Therapy, can be reduced or eliminated, and allow our immune system to fight the virus.
Sadly, too often, people are killed or rendered disabled or even become chronically ill, because of the “double whammy” of a virus infection occurring, and then the person is hit by a serious bacterial infection. Our bodies have wonderful defence systems, but fighting a war on two or more fronts at the same time, may overwhelm us.
There are many ways that Bacteriophages may help us, apart from direct application via injection, nasal spray or ointment. They can be used in bandages to reduce or prevent infections in burns case, or serious wounds, used to render catheters safe, used in special preparations on hospital staff clothing (effective for 3 weeks) or even used to render the operating room or recovery ward sterile and safe.
I will gradually explain these processes and also rendering food safe for pregnant women, babies or immune compromised people, against infections such as Listeria or e-coli based illnesses, and much, much more.
..............................................................
Love Me Love My Phages
Eat Me
The Soviet method for attacking infection that we can learn from.
By Daria Vaisman
Posted Tuesday, May 30, 2006, at 12:41 PM ETFrom: Medical Examiner_Health and medicine explained.
(Illustration Above is stylized Bacteriophage)In the 1920s and '30s, with diseases like dysentery and cholera running rampant, the discovery of bacteriophages was hailed as a breakthrough. Bacteriophages are viruses found virtually everywhere—from soil to seawater to your intestines—that kill specific, infection-causing bacteria. In the United States, the drug company Eli Lilly marketed phages for abscesses and respiratory infections. (Sinclair Lewis' Pulitzer-winning Arrowsmith is about a doctor who uses phages to prevent a diphtheria epidemic.) But by the 1940s, American scientists stopped working with phages for treatment because they no longer had reason to. Penicillin, discovered by the Scottish bacteriologist Alexander Fleming in 1928, had become widely available thanks to synthetic production and zapped infections without the expertise needed for finicky phages.
But now the equation has changed. Many kinds of bacteria have become antibiotic-resistant—prompting a few Western scientists, and patients, to travel to former Soviet Georgia to give bacteriophages for treatment a try. Phages have been used in the former Soviet Union for decades because scientists there had less access to antibiotics than their American and European counterparts did. Phages were a cheap alternative, and in Soviet clinical trials, they repeatedly stopped infections. Now in a bid for medical tourists, Georgia has opened a center in its capital, Tbilisi, which offers outpatient phage treatment to foreigners. In connection with the Eliava phage research institute, which Stalin helped set up in Tbilisi in 1923, the treatment center offers personalized cures for a host of infections the United States says it can no longer do anything about.
In 2000, the Centers for Disease Control, along with other federal agencies, warned that the world might soon return to a "pre-antibiotic era." Two million people each year now get hospital-borne bacterial infections, 1.4 million of them resistant to antibiotics and 90,000 of them lethal. One example is sepsis, the infection that sickened* Joan Didion's daughter, as Didion relates in The Year of Magical Thinking. New antibiotics are being discovered. But it takes 10 years and at least $800 million to bring an antibiotic to market, according to the Infectious Diseases Society of America. The big advantage that phages offer over antibiotics is that bacterial resistance is less of a problem. Unlike antibiotics, new phage batches can quickly be whipped up to take the place of phages to which bacteria become resistant.
The word phage comes from the Greek "to eat." A phage contains genetic material that gets injected into a virus's host. Whereas "bad" viruses infect healthy cells, phages target specific bacteria that then explode. At Eliava, phages are produced as a liquid that can be drunk or injected intravenously, as pills, or as phage-containing patches for wounds. Though few published articles in Western journals report positive clinical trials—most of the recent long-term research on phages comes out of the Soviet Union—some Western scientists say that phages are safe and that they work. "There is no evidence that phage is harmful in any way," says Nick Mann, a biology professor at the University of Warwick in England and co-director of phage R&D company Novolytics.
So, why do American patients need to go to all the way to Georgia for treatment? For starters, in their natural state phages are hard to patent, the route by which drug companies lock up future profits. The first company to spend millions of dollars to prove that a particular phage is safe could allow its competitors to capitalize on the results. As important is the difficulty of regulation. There are two ways that phages are currently used in the former Soviet Union, and both pose problems from the point of view of the Food and Drug Administration. At the Tbilisi phage center, phages are personalized: You send your bacterial sample to the lab, and it's either matched up with an existing phage or a phage is cultured just for you. In the United States, by contrast, drugs are mass produced, which makes it easier for the FDA to regulate them.
Phages are also sold over-the-counter in Georgia. People take the popular mixture piobacteriophage, for example, to fight off common infections including staph and strep. These phage mixtures are updated regularly so they can attack newly emerging bacterial strains. In the United States, the FDA would want the phages in each new concoction to be gene sequenced, because regulations require every component of a drug to be identified. To do so would entail prohibitively expensive and lengthy clinical trials.
In the early years of phage research in the United States, says former National Institutes of Health scientist Carl Merril, bacteriophages allegedly killed more people than they cured. Phages are culled from dirty, wet places—the first was found in the Ganges River—a recipe for infection unless you know what you're doing. And some kinds of phages—called lysogenic phages—are potentially dangerous, because they sometimes carry genes that cause bacteria to release toxins. So, there is reason for caution.
Despite the caveats, a number of phage biotechnology firms have recently opened up in the United States and also in countries like Canada and Israel. Phage biologists point out we know much more about phage biology now than when the viruses were first discovered. Methods of using phages for treatment, from distillation to identification, have improved significantly since then. Clinicians worldwide also report that patients using phages have had good recovery rates and minor or fleeting side effects. Evergreen State College professor Elizabeth Kutter, who collaborates closely with Eliava researchers in Georgia and heads an international phage conference each year, is working with others to find ways to commercialize phages that could sidestep some of the problems with patenting and regulation. Flu vaccine offers one instructive possibility. Like over-the-counter phages, the vaccine is updated regularly with the most recent strain of flu virus—without requiring FDA approval each time.
There are already multiple uses for phages without FDA approval. A promising area is American agriculture and livestock, which is regulated by the less stringent United States Department of Agriculture. Domestic scientists are looking at ways in which phages could kill bacteria before they cause infection (rather than fight an infection after it has begun). Alexander Sulakvelidze, an assistant medical professor at the University of Maryland and a co-founder of the phage R&D company Intralytix, awaits federal approval for a phage-based wash for meat and produce that protects against food poisoning. Vincent Fischetti, a professor at the Rockefeller Institute, is designing a phage-based enzyme solution that can be sprayed into the noses and mouths of hospital and nursing-home patients. Fischetti and researchers in Tbilisi are also experimenting with using phages to detect anthrax and cholera in the case of a terrorist attack.
Using phages to treat infections at home, on the other hand, for the moment seems unlikely. One company recently tried to open a phage center in Tijuana but was deterred by the Mexican government. Phages might be offered someday at clinics on Native American reservations, as a casino-like quirk of legislative autonomy. But for now, U.S. patients at a loss for options may decide that Tbilisi is close enough.
Correction, June 1, 2006: The original sentence stated that Didion's daughter died of sepsis. In fact, she got sick from sepsis but died later from another condition. Click here to return to the corrected sentence.
Love Me Love My Phages
................................................................
Love Me Love My Phages
Phage Therapy
Phage Therapy
(From Wikipedia, the free encyclopedia)
Phage Therapy
An important theoretical benefit of phage therapy is that bacteriophages can be much more specific than more common drugs, so can be chosen to be harmless to not only the host organism (human, animal, or plant), but also other beneficial bacteria, such as gut flora, reducing the chances of opportunistic infections. They also have a high therapeutic index, that is, phage therapy gives rise to few if any side effects, as opposed to drugs, and does not stress the liver. Because phages replicate in vivo, a smaller effective dose can be used. On the other hand, this specificity is also a disadvantage: A phage will only kill a bacterium if it is a match to the specific strain. Thus, phage mixtures are often applied to improve the chances of success, or samples can be taken and an appropriate phage identified and grown.
Phages are currently being used therapeutically to treat bacterial infections that do not respond to conventional antibiotics, particularly in the country of Georgia.[2][3][4] They tend to be more successful than antibiotics where there is a biofilm covered by a polysaccharide layer, which antibiotics typically cannot penetrate.[citation needed] In the West, no therapies are currently authorized for use on humans, although phages for killing food poisoning bacteria (Listeria) are now in use.[5]
History
Following the discovery of bacteriophages by Frederick Twort and Felix d'Hérelle[6] in 1915 and 1917, phage therapy was immediately recognized by many to be a key way forward for the eradication of bacterial infections. A Georgian, George Eliava, was making similar discoveries. He travelled to the Pasteur Institute in Paris where he met d'Hérelle, and in 1926 he founded the Eliava Institute in Tbilisi, Georgia, devoted to the development of phage therapy.In neighbouring countries including Russia, extensive research and development soon began in this field. In the USA during the 1940s, commercialization of phage therapy was undertaken by the large pharmaceutical company, Eli Lilly.
Whilst knowledge was being accumulated regarding the biology of phages and how to use phage cocktails correctly, early uses of phage therapy were often unreliable. When antibiotics were discovered in 1941 and marketed widely in the USA and Europe, Western scientists mostly lost interest in further use and study of phage therapy for some time.[7]
Isolated from Western advances in antibiotic production in the 1940s, Russian scientists continued to develop already successful phage therapy to treat the wounds of soldiers in field hospitals. During World War II, the Soviet Union used bacteriophages to treat many soldiers infected with various bacterial diseases e.g. dysentery and gangrene. The success rate was as good as, if not better than any antibiotic.[citation needed] Russian researchers continued to develop and to refine their treatments and to publish their research and results. However, due to the scientific barriers of the Cold War, this knowledge was not translated and did not proliferate across the world.[8][9]
There is an extensive library and research center at the Eliava Institute in Tbilisi, Georgia. Phage therapy is today a widespread form of treatment in neighbouring countries. For 80 years Georgian doctors have been treating local people, including babies and newborns, with phages.
As a result of the development of antibiotic resistance since the 1950s and an advancement of scientific knowledge, there is renewed interest worldwide in the ability of phage therapy to eradicate bacterial infections and chronic polymicrobial biofilm, along with other strategies.
Phages have been explored as means to eliminate pathogens like Campylobacter in raw food[10] and Listeria in fresh food or to reduce food spoilage bacteria.[11] In agricultural practice phages were used to fight pathogens like Campylobacter, Escherichia and Salmonella in farm animals, Lactococcus and Vibrio pathogens in fish from aquaculture and Erwinia and Xanthomonas in plants of agricultural importance. The oldest use was, however, in human medicine. Phages were used against diarrheal diseases caused by E. coli, Shigella or Vibrio and against wound infections caused by facultative pathogens of the skin like staphylococci and streptococci. Recently the phage therapy approach has been applied to systemic and even intracellular infections and the addition of non-replicating phage and isolated phage enzymes like lysins to the antimicrobial arsenal. However, definitive proof for the efficiency of these phage approaches in the field or the hospital is only provided in a few cases.[11]
Some of the interest in the West can be traced back to 1994, when Soothill demonstrated (in an animal model) that the use of phages could improve the success of skin grafts by reducing the underlying Pseudomonas aeruginosa infection.[12] Recent studies have provided additional support for these findings.[13]
Recently, the use of phages as delivery mechanisms for traditional antibiotics has been proposed.[14][15] The use of phages to deliver antitumor agents has also been described, in preliminary in vitro experiments for cells in tissue culture.[16]
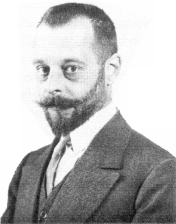
.................................................
Potential Benefits
A potential benefit of phage therapy is freedom from the severe adverse effects of antibiotics. Also it would possibly be fast-acting, once the exact bacteria are identified and the phages administered. Another benefit of phage therapy is that although bacteria are able to develop resistance to phages the resistance might be easier to overcome.
Bacteriophages are often very specific, targeting only one or a few strains of bacteria.[17] Traditional antibiotics usually have more wide-ranging effect, killing both harmful bacteria and useful bacteria such as those facilitating food digestion. The specificity of bacteriophages might reduce the chance that useful bacteria are killed when fighting an infection.
Increasing evidence shows the ability of phages to travel to a required site — including the brain, where the blood brain barrier can be crossed — and multiply in the presence of an appropriate bacterial host, to combat infections such as meningitis. However the patient's immune system can, in some cases mount an immune response to the phage (2 out of 44 patients in a Polish trial[18]). This might possibly be therapeutically significant.
Development and production is faster than antibiotics, on condition that the required recognition molecules are known.[citation needed]
Research groups in the West are engineering a broader spectrum phage and also target MRSA treatments in a variety of forms - including impregnated wound dressings, preventative treatment for burn victims, phage-impregnated sutures. Enzobiotics are a new development at Rockefeller University that create enzymes from phage. These show potential for preventing secondary bacterial infections e.g. pneumonia developing with patients suffering from flu, otitis etc..[citation needed]
Some bacteria such as multiply resistant Klebsiella pneumoniae have no non toxic antibiotics available, and yet killing of the bacteria via intraperitoneal, intravenous or intranasal of phages in vivo has been shown to work in laboratory tests.[19]
Application
Collection
In its simplest form, phage treatment works by collecting local samples of water likely to contain high quantities of bacteria and bacteriophages, for example effluent outlets, sewage and other sources.[2] They can also be extracted from corpses. The samples are taken and applied to the bacteria that are to be destroyed which have been cultured on growth medium.
The bacteria usually die, and the mixture is centrifuged. The phages collect on the top of the mixture and can be drawn off.
The phage solutions are then tested to see which ones show growth suppression effects (lysogeny) and/or destruction (lysis) of the target bacteria. The phage showing lysis are then amplified on cultures of the target bacteria, passed through a filter to remove all but the phages, then distributed.
Treatment
Phages are "bacterium specific" and it is therefore necessary in many cases to take a swab from the patient and culture it prior to treatment. Occasionally, isolation of therapeutic phages can typically require a few months to complete, but clinics generally keep supplies of phage cocktails for the most common bacterial strains in a geographical area.
Phages in practice are applied orally, topically on infected wounds or spread onto surfaces, or used during surgical procedures. Injection is rarely used, avoiding any risks of trace chemical contaminants that may be present from the bacteria amplification stage,and recognizing that the immune system naturally fights against viruses introduced into the bloodstream or lymphatic system.
The direct human use of phage might possibly be safe; suggestively, in August 2006, the United States Food and Drug Administration approved spraying meat with phages. Although this initially raised concerns since without mandatory labeling consumers won't be aware that meat and poultry products have been treated with the spray,[20] it confirms to the public that, for example, phages against Listeria are generally recognized as safe (GRAS status) within the worldwide scientific community and opens the way for other phages to also be recognized as having GRAS status.
Phage therapy has been attempted for the treatment of a variety of bacterial infections including: laryngitis, skin infections, dysentery, conjunctivitis, periodontitis, gingivitis, sinusitis, urinary tract infections and intestinal infections, burns, boils, etc.[2] - also poly-microbial biofilms on chronic wounds, ulcers and infected surgical sites.[citation needed]
In 2007, Phase 2a clinical trials have been reported at the Royal National Throat, Nose and Ear Hospital, London for Pseudomonas aeruginosa infections (otitis).[21].[22][23] Documentation of the Phase-1 and Phase-2a study is not available at present.
Phase 1 clinical trials are underway in the South West Regional Wound Care Center, Lubbock, Texas for an approved cocktail of phages against bacteria, including P. aeruginosa, Staphylococcus aureus and Escherichia coli (better known as E. coli).[citation needed]
Reviews of phage therapy indicate that more clinical and microbiological research is needed to meet current standards.[24]
Distribution
Phages can usually be freeze dried and turned into pills without materially impacting efficacy.[2] In pill form temperature stability up to 55 C, and shelf lives of 14 months have been shown.[citation needed] Other forms of administration can include application in liquid form. These vials are usually best kept refrigerated.[citation needed]
Oral administration works better when an antacid is included, as this increases the number of phages surviving passage through the stomach.[citation needed]
Topical administration often involves application to gauzes that are laid on the area to be treated.[citation needed]
Obstacles
General
The host specificity of phage therapy may make it necessary for clinics to make different cocktails for treatment of the same infection or disease because the bacterial components of such diseases may differ from region to region or even person to person. Such a process would make it difficult for large scale production of phage therapy. Additionally, patent issues (specifically on living organisms) may complicate distribution for pharmaceutical companies wishing to have exclusive rights over their "invention"; making it unlikely that a for-profit corporation will invest capital in the widespread application of this technology.In addition, due to the specificity of individual phages, for a high chance of success, a mixture of phages is often applied. This means that 'banks' containing many different phages are needed to be kept and regularly updated with new phages, which makes regulatory testing for safety harder and more expensive.
Some bacteria, for example Clostridium and Mycobacterium, have no known therapeutic phages available as yet.
To work, the virus has to reach the site of the bacteria, and viruses do not necessarily reach the same places that antibiotics can reach.
Funding for phage therapy research and clinical trials is generally insufficient and difficult to obtain, since it is a lengthy and complex process to patent bacteriophage products. Scientists comment that 'the biggest hurdle is regulatory', whereas an official view is that individual phages would need proof individually because it would be too complicated to do as a combination, with many variables. Due to the specificity of phages, phage therapy would be most effective with a cocktail injection, which are generally rejected by the FDA. Researchers and observers predict that for phage therapy to be successful the FDA must change its regulatory stance on combination drug cocktails.[25] Public awareness and education about phage therapy are generally limited to scientific or independent research rather than mainstream media.[26]
The negative public perception of viruses may also play a role in the reluctance to embrace phage therapy.[27]
Safety
Phage therapy is generally considered safe. As with antibiotic therapy and other methods of countering bacterial infections, endotoxins are released by the bacteria as they are destroyed within the patient (Herxheimer reaction). This can cause symptoms of fever, or in extreme cases toxic shock (a problem also seen with antibiotics) is possible.[28] Janakiraman Ramachandran, a former president of AstraZeneca India who 2 years ago launched GangaGen Inc., a phage-therapy start-up in Bangalore,[29] argues that this complication can be avoided in those types of infection where this reaction is likely to occur by using genetically engineered bacteriophages; which have had their gene responsible for producing endolysin removed. Without this gene the host bacterium still dies but remains intact because the lysis is disabled. On the other hand this modification stops the exponential growth of phages, so one administered phage means one dead bacterial cell.[4] Eventually these dead cells are consumed by the normal house cleaning duties of the phagocytes, which utilise enzymes to break the whole bacterium and its contents down into its harmless sub-units of proteins, polysaccharides and lipids.[30]
Care has to be taken in manufacture that the phage medium is free of bacterial fragments and endotoxins from the production process.
Lysogenic bacteriophages are not generally used therapeutically. This group can act as a way for bacteria to exchange DNA, and this can help spread antibiotic resistance or even, theoretically, can make the bacteria pathogenic (see Cholera).
The lytic bacteriophages available for phage therapy are best kept refrigerated but discarded if the pale yellow clear liquid goes cloudy.
Cultural references
- The novel Arrowsmith used phage therapy as a plot point.[31][32][33]
See also
References
- ^ McAuliffe et al. "The New Phage Biology: From Genomics to Applications" (introduction) in Mc Grath, S. and van Sinderen, D. (eds.) Bacteriophage: Genetics and Molecular Biology Caister Academic Press ISBN 978-1-904455-14-1.reprint
- ^ a b c d BBC Horizon: Phage - The Virus that Cures
- ^ Parfitt T (2005). "Georgia: an unlikely stronghold for bacteriophage therapy". Lancet 365 (9478): 2166–7. doi: . PMID 15986542.
- ^ a b Thiel, Karl (January 2004). "Old dogma, new tricks—21st Century phage therapy". Nature Biotechnology (London UK: Nature Publishing Group) 22 (1): 31–36. doi: . ISSN 1087-0156. http://www.nature.com/nbt/journal/v22/n1/full/nbt0104-31.html. Retrieved on 15 December 2007.
- ^ Pirisi A (2000). "Phage therapy—advantages over antibiotics?". Lancet 356 (9239): 1418. doi: . PMID 11052592.
- ^ Shasha SM, Sharon N, Inbar M (2004). "[Bacteriophages as antibacterial agents]" (in Hebrew). Harefuah 143 (2): 121–5, 166. PMID 15143702.
- ^ Hanlon GW (2007). "Bacteriophages: an appraisal of their role in the treatment of bacterial infections". Int. J. Antimicrob. Agents 30 (2): 118–28. doi: . PMID 17566713. http://linkinghub.elsevier.com/retrieve/pii/S0924-8579(07)00203-8.
- ^ "Stalin's Forgotten Cure" Science (magazine) 25 October 2002 v.298 [www.sciencemag.org]reprint
- ^ Summers WC (2001). "Bacteriophage therapy". Annu. Rev. Microbiol. 55: 437–51. doi: . PMID 11544363.
- ^ Mangen MJ, Havelaar AH, Poppe KP, de Wit GA (2007). "Cost-utility analysis to control Campylobacter on chicken meat: dealing with data limitations". Risk Anal. 27 (4): 815–30. doi: . PMID 17958494. http://www.blackwell-synergy.com/openurl?genre=article&sid=nlm:pubmed&issn=0272-4332&date=2007&volume=27&issue=4&spage=815.
- ^ a b Mc Grath S and van Sinderen D (editors). (2007). Bacteriophage: Genetics and Molecular Biology (1st ed. ed.). Caister Academic Press. ISBN 978-1-904455-14-1 . http://www.horizonpress.com/phage.
- ^ Soothill JS (1994). "Bacteriophage prevents destruction of skin grafts by Pseudomonas aeruginosa". Burns 20 (3): 209–11. doi: . PMID 8054131.
- ^ McVay CS, Velásquez M, Fralick JA (2007). "Phage therapy of Pseudomonas aeruginosa infection in a mouse burn wound model". Antimicrob. Agents Chemother. 51 (6): 1934–8. doi: . PMID 17387151. http://aac.asm.org/cgi/pmidlookup?view=long&pmid=17387151.
- ^ Yacoby I, Bar H, Benhar I (2007). "Targeted drug-carrying bacteriophages as antibacterial nanomedicines". Antimicrob. Agents Chemother. 51 (6): 2156–63. doi: . PMID 17404004. http://aac.asm.org/cgi/pmidlookup?view=long&pmid=17404004.
- ^ Yacoby I, Shamis M, Bar H, Shabat D, Benhar I (2006). "Targeting antibacterial agents by using drug-carrying filamentous bacteriophages". Antimicrob. Agents Chemother. 50 (6): 2087–97. doi: . PMID 16723570. http://aac.asm.org/cgi/pmidlookup?view=long&pmid=16723570.
- ^ Bar H, Yacoby I, Benhar I (2008). "Killing cancer cells by targeted drug-carrying phage nanomedicines". BMC Biotechnol. 8: 37. doi: . PMID 18387177. http://www.biomedcentral.com/1472-6750/8/37.
- ^ Duckworth DH, Gulig PA (2002). "Bacteriophages: potential treatment for bacterial infections". BioDrugs 16 (1): 57–62. PMID 11909002.
- ^ "Non-antibiotic therapies for infectious diseases." by Christine F Carson, and Thomas V Riley Communicable Diseases Intelligence Volume 27 Supplement - May 2003 Australian Dept of health website
- ^ [1]
- ^ http://www.forbes.com/business/healthcare/feeds/ap/2006/08/18/ap2959720.html
- ^ "Press & News". Retrieved on 2007-12-13.
- ^ "biocontrol.ltd.uk". Retrieved on 2007-12-13.
- ^ "biocontrol-ltd.com". Retrieved on 2008-04-30.
- ^ " "Phage therapy: the Escherichia coli experience" by Harald Brüssow in Microbiology (2005) v. 151, p.2133-2140. publisher site
- ^ Thiel K (2004). "Old dogma, new tricks—21st Century phage therapy". Nat. Biotechnol. 22 (1): 31–6. doi: . PMID 14704699.
- ^ Brüssow, H 2007. Phage Therapy: The Western Perspective. in S. McGrath and D. van Sinderen (eds.) Bacteriophage: Genetics and Molecular Biology, Caister Academic Press, Norfolk, UK. ISBN 978-1-904455-14-1
- ^ Verbeken G, De Vos D, Vaneechoutte M, Merabishvili M, Zizi M, Pirnay JP (2007). "European regulatory conundrum of phage therapy". Future Microbiol 2 (5): 485–91. doi: . PMID 17927471. http://www.futuremedicine.com/doi/abs/10.2217/17460913.2.5.485.
- ^ Evergreen PHAGE THERAPY: BACTERIOPHAGES AS ANTIBIOTICS
- ^ Stone, Richard. "Stalin's Forgotten Cure." Science Online 282 (25 October 2002).
- ^ Fox, Stuart Ira (1999). Human Physiology -6th ed.. McGraw-Hill. pp. : 50,55,448,449. ISBN 0-697-34191-7. http://www.mhhe.com.
- ^ Summers WC (1991). "On the origins of the science in Arrowsmith: Paul de Kruif, Felix d'Herelle, and phage". J Hist Med Allied Sci 46 (3): 315–32. doi: . PMID 1918921.
- ^ "Phage Findings - TIME". Retrieved on 2007-12-13.
- ^ "SparkNotes: Arrowsmith: Chapters 31–33". Retrieved on 2007-12-13.
Retrieved from "http://en.wikipedia.org/wiki/Phage_therapy"
Illustrations below are Frederick Twort and Felix d'Hérelle
Love Me Love My Phages
...........................................................................................
Page 1
Love Me Love My PhagesCholera epidemics and the role bacteriophages may play in ending them
Cholera epidemics (caused by the bacterium Vibrio cholerae) cause widespread illness and death in developing countries. The Ganges Delta region of Bangladesh and India, for example, suffers two cholera epidemics each year. ICDDR,B researchers and Harvard Medical School have therefore been working to identify what factors trigger and end these seasonal epidemics. Results suggest that bacteriophages (viruses that attack bacteria) may play a key role.
"Researchers will use their improved understanding of the interactions among hosts,
V. cholerae, and cholera-killing bacteriophages to identify new ways of preventing
cholera epidemics"
V. cholerae, and cholera-killing bacteriophages to identify new ways of preventing
cholera epidemics"
During a three-year study of patients in ICDDR,B’s Dhaka hospital, researchers confirmed that the number of cholera patients (which varied seasonally) often coincided with the presence of disease-causing V. cholerae strains in water samples. They also showed that, during epidemic-free periods, water supplies typically contained cholera-killing phages but no viable bacteria.
Importantly, researchers also found that the phage peak in water samples coincided with a rise in the number of phages found in the excrement of cholera patients. So, it seems that the epidemics are ended by phages which amplify in people with cholera—these then attack the cholera bacteria once they are excreted and enter water supplies. This may well explain why the seasonal cholera epidemics that occur in Bangladesh are self-limiting.
Before the development of modern antimicrobials, phage therapy was considered a feasible option for the treatment of bacterial infection. The study team will therefore use their improved understanding of the interactions among hosts, V. cholerae,and cholera phages to identify new ways of preventing cholera epidemics
Page 1
|
.........................................................................................
The Cocktail That Cures
THE HINDU
Online edition of India's National Newspaper
Sunday, November 26, 2000
HOW far away are we from a return to time when people die from a sore throat? At a press conference on May 23, 1997, scientists finally acknowledged the arrival of untreatable bacteria they had feared for years - bacteria that resist antibiotics. Drugs which have kept us safe for 50 years were beginning to fail, they said.
Today, superbugs look triumphant and this is a serious situation. Over the last five years, scientists have clearly seen a change in their ability to tackle what should have been easily treatable infections, because bacteria are developing the ability to resist antibiotics. And the more antibiotics we use, the more resistant bacteria become.
Every year, more than five million people die from infections that do not respond to antibiotics. Things are going to get worse. Staphylococcus, one of the most dangerous bacteria, now has only one antibiotic to keep it in check - Vancomycin.
This year, Japanese doctors saw the world's first case of infection with Vancomycin-resistant staphylococcus - a baby boy in hospital for major heart surgery. When antibiotics failed, doctors had to pour strong disinfectants directly into the wound on his chest. It was quite shocking because the outcome of that infection was quite hideous. The patient suffered a lot.
Terrible bacteria will inevitably spread, and when they do, being in hospital even for minor surgery, or a hip operation or to have a baby, could be lethal. If even the smallest wound becomes infected, bacteria would most probably kill you. It would be very hard to conduct major surgery that we have got used to. Transplants, cancer chemotherapy, are all dependent on the ability to kill off bacteria which may infect patients. We will lose all that.
It is hard to understand why no new drugs have been developed to save mankind. Pharmaceutical companies should have come to grips with the situation. But they thought that that was not profitable a decade ago. Now, it is too late.
Even today, drug companies do not promise a new class of antibiotics for at least 10 years and they may never discover one. They have already exhausted traditional chemistry and computer drug design. Today, they analyse the genes of the bacteria, hoping to find new strategies. But the frightening truth is that no fundamentally new antibiotic has been discovered for more than 30 years.
One begins to worry that we are indeed moving into what some people have called the post-antibiotic era, where bacteria are supreme.
But there is a major remedy that kills even the most resistant bacteria.
Unknown to the rest of the world, in a small country in the heart of the Caucasus Mountains, south of Moscow and north of Turkey, scientists in the Republic of Georgia may have the answer against superbugs.
In the central hospital in the Republic of Georgia, a former Soviet State, patients recover like elsewhere. They are weak and vulnerable and would probably die if they caught an infection.
The doctors here know that there is a problem. It has become the breeding ground for particularly nasty bacteria, which they suspect are resistant to all the antibiotics they have. They are taking samples from every surface in the ward to know exactly what they are up against. Some of the samples have traces of staphylococcus strains.
Anywhere else in the world, this would be a death sentence. But here in Georgia, the doctors are not too worried because they have an answer - a unique medicine that still works on antibiotic-resistant infections. The same amazing potion is used to treat both patients and wards. It has a remarkable effect on bacteria.
Two flasks contain bacteria. A few drops of the Georgian medicine have been added to one and it has a magical effect. The bacteria have all been killed. This astonishing effect is caused by something we usually fear - a virus, and one that comes from sewage. Says Dr. Teimuraz Chanishvili, Institute of Bacteriophage, Tbilisi, "This happened 51 years ago. It was the first experiment I did here. I took the culture of bacteria and added sewage to it, just ordinary sewage water from the drains. I first saw this a long time ago, when I was still a student. We were all very enthusiastic about it."
The first to spot what happened was an irascible French-Canadian called Felix d'Herelle. In 1917, he suggested that the viruses which killed bacteria in the bottles could be used to treat disease. An ardent Communist, d'Herelle was enticed to Russia by Stalin, who wanted this magic medicine for his army. And in the Finnish war of the 1930's, and even in World War II, it was used to protect the Red Army from the dysentery and gangrene that plagued the battlefield.
With Stalin's blessing, d'Herelle founded an institute in Tbilisi, the capital of Georgia, that was dedicated to the study of these magical viruses and the way they cure infections. D'Herelle named these healing viruses as bacteriophage, which means "bacteria eaters". There is a phage to kill every kind of bacteria. Just as bacteria attack people, these tiny viruses attack them, and they are found wherever bacteria thrive, most often in sewage.
Phage have an extraordinary structure. Their bulk is a head in which their genes are stored. They have six legs or filaments, which attach themselves to the bacteria and a tail that works like a hypodermic syringe to infect it with their genes. Inside the bacteria, the phage viruses grow and multiply. Sometimes as many as 5,000 grow in a single cell.
When the new phage burst out, they kill the bacteria and then each goes on to find another victim. Each phage only grows on a particular kind of bacteria. That is why when we talk of Staphylococcus phage, it is known that it reproduces only on Staphylococcus.
But being specific makes phage tricky to work with. Over the years, the institute has trained specialists to find phage, grow them and turn them into medicine. In the 1970s when antibiotic- resistant bacteria became a serious problem in Soviet hospitals, phage became the saviours.
A decree was issued that all bacteria resistant to antibiotics and local phages must be sent to the institute, where a new preparation was made. It was very difficult to organise, but everything was done under one roof. The centralised Soviet system was ideal for the labour-intensive work needed to make phage effective. Together, Communism, phage and the institute thrived.
Phage medicine had its heyday in the 1980s. It was manufactured in factories across the Soviet Union and the Tbilisi Institute. At that time, Teimuraz Chanishvili and Amiran Meipariani ran the institute.
Remembering those days they say, "We used to inject phage into one, two, three, four vats. There were 500 litres in each vat, and remember we had to take orders on top of that. We produced tablets and bottles. We made phages for the Soviet Union. They were not only for dysentery but typhoid and salmonella too. Most were for intestinal infections. Phages are our daily bread. We have devoted our lives to them. The prospects for phage are tremendous. There is no question that phage medicine can be extremely effective."
An old woman recalls how phage helped cure her son, "My son became ill when he was young. They checked his throat and nose and found Staphylococcus. I went to the institute and they gave him phage and cured him. Phage medicine is a wonderful thing. It works against dysentery. They even give you phage in an enema."
Today, when people in Georgia get an infection, they take antibiotics at times. Often the doctors prescribe phage. There is a pharmacy on the grounds of the Tbilisi Institute. Everyday, people who choose to use phage, rather than antibiotics, go there to have their infections diagnosed and to pick up prescriptions for medicines, tablets and creams made of phage.
They rub the healing viruses into their wounds, drink them for a bad stomach, or swirl a solution in their months to cure a gum infection. A woman developed gangrene in a wound on her thumb. The doctors cut away most of the infected tissue, the rest they treated with phage. If the resistant bacteria rife in the hospital got into this woman's wound, it would have caused fatal blood poisoning. Phage does not work well in the bloodstream.
Being a virus, it is fought off by the immune system. But the surgeons make sure infection does not take hold in the first place, by using phage to sterilise the room and equipment as well as the wound. The doctor in attendance says, "We think that the phage that was used during the first operation helped the wound to be in such good condition." Phage works wonders in Georgia and given the chance, it could do the same everywhere."
A unique library of phage medicine exists in Tblisi. It is a national treasure, built up over 50 years when problem bacteria was sent here from all across the Soviet Union. Phages were found to fight every new infection and then they and the bacteria they killed were stored separately, for future research. It is the biggest collection of phage medicine in the world.
Phage therapy has been so successful in the Soviet Union for so long, that it is hard to understand why people in the West have never even heard of it. To scientists like Dr. Chanishvili, it seems incredible that this medicine, once used daily right across the Soviet Union, has been ignored by the West. But the reasons are woven tightly into history.
In Britain today, very few people know anything about phage therapy, but those that do are clear about how it became discredited in the West in the 1930s.
Some of the claims made for some of these bacterial phages which are isolated were, quite frankly, barmy. There was one commercial preparation, for example, called Enterofagos, which supposedly had miraculous powers against both herpes infections and eczema.
These claims were not fraudulent. In fact, a lack of understanding plagued most phage work done around the world in the early days. In India in 1935, even the British army tried it out on the local population during a cholera epidemic.
It has to be said that some of the clinical trials that were carried out were of exceedingly poor quality. For example, in some of the early work on choleraphage in the 1920s and the 1930s, there were no control groups. So it was impossible to see whether the phage had worked.
And some of the trials consisted merely in pouring bacteriophage down drinking wells in a village and see whether it had any effect with no understanding of dosage or the mechanisms whereby bacteria produced the diseases.
Some of the problems with early studies though is the best phages for the job were not selected. They did not check whether the organism was sensitive to the phage. By 1941, phage was still thought to be too unreliable to be useful by researchers in the West. W. When powerful antibiotics arrived a few years later, it was quickly forgotten. Meanwhile, unknown to the outside world, the Georgian scientists went on working.
Research that proves the worth of phage medicine was published by the Georgian scientists, but only in the Soviet Union.
And even today, it is ignored because of a strange phenomenon that is true across the world of science. The articles were published either in Russian or in Georgian. Thus, language has proved to be the final barrier. So, perhaps the real problem was not because the science was bad, but because the findings could not be read. And so the West could not get acquainted with it.
Modern medicine faces a crisis as new strains of antibiotic- resistant bacteria threaten advanced treatments and intensive care. But there is an unlikely saviour - a virus derived from sewage that can kill bacteria. To learn more about this unlikely saviour watch "Vital Breakthroughs", Sunday, from 7 to 8 p.m., on the Discovery Health Block, only on Discovery Channel.
Information and picture courtesy: Discovery Channel
Love Me Love My Phages
......................................................
Phage Therapy in Agriculture and Animal Husbandry
It may surprise many people just how important that the study of Bacteriophages and the implementation of Phage Therapy in the growing of crops, and in looking after animals or even fish, as like all living things they take the same risks as we do, and can infect us, or ev en wipe out civilisations with huge crop failures.
The following article deals the following important issues, among many others.
http://www.adsa.org/discover/7thDISCOVERProg4-8-03_files/INTERPRETIVE%20SUMMARIES/BrabbanADSAphageabstract.htm
Phage Therapy in Agriculture and Animal Husbandry
It may surprise many people just how important that the study of Bacteriophages and the implementation of Phage Therapy in the growing of crops, and in looking after animals or even fish, as like all living things they take the same risks as we do, and can infect us, or ev en wipe out civilisations with huge crop failures.
The following article deals the following important issues, among many others.
1) Phage to control plant diseases such as bacterial spot on tomatoes and Erwinia infections of fruit trees (fire blight) and root crops (soft rot).
2) Phage to treat animal disease such as E. coli respiratory infections in chickens, furunculosis (Aeromonas salmonicida) in fish, and mastitis in cattle.
3) Phage to control human food-borne pathogens, such as Salmonella in fowl and Escherichia coli O157:H7 in cattle, and Listeria during food processing.
Phage Therapy: new methods for the potential eradication of E. coli O157 in livestock.
Andrew Brabban1, Raul Raya1, Todd Callaway2, and Elizabeth Kutter1. 1The Evergreen State College, Olympia WA., and 2USDA Agricultural Station, College Station, TX.
Since the advent of antibiotics, both the health care and agriculture sectors have relied heavily on them to control bacterial pathogens. However, increasing levels of antibiotic resistance have reduced the efficacy of many current therapies, prompting legislation that has reduced the use of antibiotics in animals. This has led researchers to seek fresh ideas. Bacteriophage therapy is one “old” idea undergoing a renaissance, with the potential to resolve the antibiotic predicament we find ourselves in today. Lytic bacteriophages are viruses that attach to specific bacterial surface receptors, inject their DNA, and express genes that lead to the synthesis of new phages. The process ends with the programmed lysis (death) of the host and the release of dozens or hundreds of new phages. The use of phages as antimicrobial agents has a number of advantages over other methods. Phages are highly specific allowing for the removal of the targeted microorganisms from a mixed population. Unlike antibiotics that decay over time and distance, phage numbers actually increase working their way deeper into pockets of infestation. Further phage are living entities that adapt and evolve. As phage can also pass from host to host, they have the potential to establish an infectious cure. Interest in agricultural applications is now rapidly expanding in 3 major areas;
1) Phage to control plant diseases such as bacterial spot on tomatoes and Erwinia infections of fruit trees (fire blight) and root crops (soft rot).
2) Phage to treat animal disease such as E. coli respiratory infections in chickens, furunculosis (Aeromonas salmonicida) in fish, and mastitis in cattle.
3) Phage to control human food-borne pathogens, such as Salmonella in fowl and Escherichia coli O157:H7 in cattle, and Listeria during food processing.
Today I will talk about our most recent efforts to control Escherichia coli O157:H7; a pathogen that can produce severe diarrhea, kidney damage and death in humans. In the U.S., >70,000 illnesses and 60 deaths are reported yearly with most cases traced back to livestock contamination - drinking water, milk, produce or ground beef.. Livestock show no signs of illness and the levels are generally low, making contaminated animals hard to identify. We have developed a promising way of reducing O157:H7 concentrations in livestock through bacteriophages CEV1, CEV2 and CEV3. Phage CEV1 was isolated from the feces of sheep resistant to gastrointestinal colonization by O157:H7 strains that had previously been used as standard gut colonizers. Phages CEV1 and CEV2 infect >90% of the O157:H7 strains tested, and a small number other strains. Preliminary trials of CEV1 and CEV2 to evaluate their use as a potential management strategy in vitro and in vivo have been highly successful. In model systems reflecting the cow/sheep gut, CEV1 eliminated the two virulent 0157:H7 strains in 11 days. Initial animal studies have shown the phage have great promise as an orally administered treatment; treated sheep showed a substantial reduction in intestinal levels of O157:H7 in with a single dose of CEV1 within 2 days. Further reduction was seen with a cocktail of CEV1 and CEV2, as well as in those sheep naturally carrying phage CEV2. These results indicate the protective effect of bacteriophages against E. coli O157:H7. We hope this approach will significantly contribute to the important job of creating a safer human food supply for the 21st Century.
Dr. Andrew D. Brabban, Lab I, The Evergreen State College, Olympia, WA 98503. Tel: 360-867-6157, Fax: 360-867-6791. brabbana@evergreen.edu, http://academic.evergreen.edu/b/brabbana/ http://www.evergreen.edu/phage/home.htmL
------------------------------------
Archivum Immunologiae et Therapiae Experimentalis, 1999, 47, 267–274
PL ISSN 0004-069X
Review
Phage Therapy: Past History and Future Prospects
RICHARD M. CARLTON
Exponential Biotherapies, Inc., 150 Main Street, Port Washington, NY 11050, USA
PL ISSN 0004-069X
Review
Phage Therapy: Past History and Future Prospects
RICHARD M. CARLTON
Exponential Biotherapies, Inc., 150 Main Street, Port Washington, NY 11050, USA
- Abstract. Bacterial viruses (bacteriophages, also called “phages”) can be robust antibacterial agents in vitro. However, their use as therapeutic agents, during a number of trials from the1920s to the 1950s, was greatly handicapped by a number of factors. In part, there were certain limitations inherent in phage physiology (e. g. narrow host range, and rapid clearance from the body); in part there were technological limitations in the era (e.g. lysogeny not yet discovered); but the greatest limitation was the highly inadequate scientific methodologies used by practitioners at the time (e.g., their failure to conduct placebo-controlled studies, to remove endotoxins from the preparations, and to re-confirm phage viability after adding sterilizing agents to the preparations). In recent years, well-controlled animal models have demonstrated that phages can rescue animals from a variety of fatal infections, while non-controlled clinical reports published in Eastern Europe have shown that phages can be effective in treating drug-resistant infections in humans. This encouraging data, combined with the fact that drug-resistant bacteria have become a global crisis, have created a window of opportunity for phage therapy to be tested anew, this time using modern technologies and placebo-controlled designs. If successful, it can be used as a stand-alone therapy when bacteria are fully resistant to antibiotics, and as a valuable adjunct to antibiotics when the bacteria are still susceptible. -------------------------------------------------------------------------
Phage Therapy - Past History and Future Prospects
A number of reviews provide details on phage therapy’s ascent and decline in the historical era 1–3, 13, 14.
We will summarize some of the more salient features of this history.
Phages were discovered in 1915 by British microbiologist Felix Twort, and, independently in 1917, by French-Canadian microbiologist Felix d’Hérelle. Twort did not pursue his discovery, whereas d’Hérelle systematically investigated the nature of bacteriophages and explored their ability to function as therapeutic agents 5, 6.
D’Hérelle received a fair measure of fame for his discovery. He was appointed Professor of Protobiology at Yale University Medical Center, and was also on the staff of the Pasteur Institute. In 1931 he gave a series of monthly lectures on phage therapy to the New YorkAcademy of Medicine. He established phage therapy centers in several countries, including the U. S., France, and Soviet Georgia. A fictionalized account of his work was depicted in rrowsmith, the Pulitzer-prize winning novel by Sinclair Lewis.
There are many attributes of phages (see Table 1) that would tend to favor a positive outcome in therapy. Despite these attributes of phages, there were so many problems with the way phage therapy was practiced in the historical era that, by the time antibiotics were introduced in mid-century, it was already in sharp decline in the West. The investigators who developed antibiotics did not make the kinds of mistakes exhibited by the early phage investigators.
Problem 1. Host range
The issue. Phages tend to have a relatively narrow host range, posing certain disadvantages. A disadvantage is that one should administer only those phage strains shown to be strongly lytic for the bacterial strain infecting the given patient. If the patient’s condition is too critical to take the time required for this matching, then one should use a grouping (a panel) of phages, where each of the phages therein has a broad-enough host range that most strains of the bacterial target are likely to be targeted. In his lectures to the New York Academy of Medicine in 1931, d’Hérelle cited the reports of other colleagues whose initial trials used phages “off the shelf” (without being shown to be virulent for the bacteria infecting the patient) and had negative outcomes, but who did match the phage to the bacteria in subsequent trials and obtained positive outcomes.
The solution. 1) Screen the bacteria infecting a given patient against a panel of phages, to ensure that one of the phage strains will be lytic (analogous to the “culture and sensitivity test” that physicians should perform; and 2) develop “multivalent” phages that lyse all or most of the bacterial strains within a given species of pathogen.
The antibiotics in use tend to be broad spectrum, thereby provoking resistance in several species and genera of bacteria (in addition to the one targeted) . Exponential growth in numbers, so that the “drug” makes more of itself at the site of infection, where it is needed.
268 R. M. Carlton: Phage Therapy in the Past and Future
The issue. Injection of even minute amounts of endotoxin and other bacterial debris can be fatal to patients. Unfortunately, many of the phage preparations used by practitioners in the historical era were crude lysates. When these preparations were injected i.v., i.p., and in some cases even intrathecally, any beneficial effect of the phages would likely have been counteracted by illness and deaths resulting from the endotoxin.
The solution. Modern technology allows density centrifugation, banding, and other methods of purification.
The solution: Sterile filtration. If chemical agents must be used, reiterate the preparation over time to ensure that the phage remain viable.
Problem 4. Rapid clearance of phages
The issue. In fairness to phage investigators in the historical era, at the time it was not an accepted practice, in any discipline, to conduct pharmacokinetic studies. However, had the early phage investigators conducted such studies, they would have discovered that bacteriophages (being foreign proteins) tend to be rapidly cleared from the circulation. This clearance problem was first documented by Merril and his colleagues in 1973 who injected high titers of phage lambda into non-immune germ-free mice. They discovered that the phages were rapidly cleared by the spleen, liver and other filtering organs of the reticulo-endothelial system (RES)7.
This was a seminal observation, given Gunther Stent’s widely-accepted statement that one of the principal reasons phages had failed as a therapeutic was their supposed inactivation by pre-existing antibodies to them. However, any clearance of the phages from the bloodstream of the germ-free animals used by Merril and his group (ref.7) would not be due to antibodies, since those animals had never previously been exposed to bacteria or bacteriophages (and so would not have antibodies). Moreover, the phages in Merril’s experiment remained viable in the spleens of these animals over a period of several days, indicating that they were neither neutralized by antibody nor engulfed by macrophages. Rather, they appeared to have been passively entrapped in (sequestered by) these filtering organs. Such trapped phages would be unavailable to reach bacteria.
The solution. The author of this review collaborated with investigators at the U.S. National Institutes of Health (MERRIL et al.11) in the development of a method to isolate and amplify phage strains that are cleared at a slower rate. We reasoned that in all species of phage, minor variations in coat proteins might be present that would enable some variants to be less easily recognized by the RES organs and to thereby remain in the circulation for longer periods of time than the “average” wild-type phage. In this “serial passage” method, the wild-type preparation is injected into an animal, and then blood samples are taken at progressively longer time points. Any phages found in the blood sample are grown to high titer and reinjected. Through iterative rounds of passage, one can amplify the long-circulating strains being isolated. U.S. and PCT patents have been granted on this method.
As predicted, these long-circulating phages were far superior to the wild-types from which they were derived, in terms of rescuing animals from an otherwise-fatal fulminant bacteremia: 1) with no treatment, all animals were dead within 48 h; 2) treatment with the wild-type phages prevented death, but the animals became critically ill (a human with such degrees of illness would be in the intensive care unit); and 3) in contrast, with administration of the long-circulating phage strain, the only sign of illness seen was mild lethargy. These results were published in the Proceedings of the National Academy of Sciences (ref. 11), and were accompanied by a Commentary by Nobel laureate Dr. JOSHUA LEDERBERG 8.
Problem 5. Lysogeny
The issue. It was not until the late 1950s that Lwoff demonstrated the ability of some phage genomes to integrate into the bacterial chromosome as “prophages.”After a period of time (up to days or weeks, or longer), such prophages can enter the lytic cycle, and will thus appear as plaques on a bacterial lawn. It is likely that some phage therapy trials in the historic era had a negative outcome due to the inadvertent use of phage strains that, being lysogens, could not provide the rapid lysis and exponential growth in numbers that are needed for full efficacy.
The solution. Use only phages that are lytic; sequence phages that are strong candidates for clinical trials, looking for (among other things) homologies to known genes of lysogeny.
Problem 6. Anti-phage antibodies
The solution. In treating chronic or recurrent infections it may be possible to administer a higher dose of phage, to compensate for those that are rendered non-viable by interaction with neutralizing antibodies. In any case, the types and titers of antibodies that develop should be systematically studied in humans.
Problem 8. The scientific style of phage investigators in the historical era, D’Hérelle’s failure to conduct placebo-controlled studies, even on chickens, is an important example of his style. This story is a notable example of the negative impact an investigator’s personality can have on the outcome of a discovery, and d’Hérelle’s style contrasts sharply to the strongly positive influence that other scientists (such as Pasteur) have had on the outcomes of their discoveries. Whereas Pasteur excelled at conceiving of definitive experiments, and was persuasive in style, d’Hérelle failed to conduct definitive experiments, and was antagonistic rather than persuasive. For example, d’Hérelle maintained to the end that phages are the sole mechanism of defense against bacterial infection.
While he may have been correct in his view that epidemics can sometimes be checked by the spontaneous appearance of a lytic strain of phage, nevertheless he was incorrect in categorically dismissing the discoveries of Nobel laureates Metchnikoff and Ehrlich, who had shown that cellular elements (white blood cells) and humoral elements (antibodies and complement) constitute the innate host defenses against infection. D’Hérelle was afforded many opportunities to integrate his discovery with those of Metchnikoff and Ehrlich, but refused to the end (see below).
D’Hérelle retreated from attacks by Bordet and others, and moved to Soviet Georgia in the 1930s (see ref. 13). An ardent communist, he dedicated the last of his published treatises to Josef Stalin. He was in Paris at the outbreak of World War II, refused to offer his skills with phage therapy to the Germans*, and spent the occupation years in prison. By the time of the liberation his health had been broken. He was invited to a post-War international scientific symposium, where colleagues made a last effort to see if they could help him bridge the gulf. He persisted in his belief that phages were the body’s sole mechanism of defense against bacteria (“Ce n’est que la phage…”), and he died in isolation in 1949. Surely the prospects of phage therapy in the historical era would have been better served if d’Hérelle had possessed some of the personality traits and scientific style of Pasteur.
The criteria of cure were cessation of suppuration and,where applicable, complete closure of wounds/fistulae (many of which had been draining for months). These investigators administer phages orally, because they are aware of the hazards of administering them parenterally (not all of the bacterial debris has been removed). They pre-treat the patients with antacids and gelatin in order to protect the phages from destruction by gastric acidity. These same investigators have published evidence that phages administered orally to humans in this manner do in fact reach the bloodstream 26.
We will summarize some of the more salient features of this history.
Phages were discovered in 1915 by British microbiologist Felix Twort, and, independently in 1917, by French-Canadian microbiologist Felix d’Hérelle. Twort did not pursue his discovery, whereas d’Hérelle systematically investigated the nature of bacteriophages and explored their ability to function as therapeutic agents 5, 6.
D’Hérelle received a fair measure of fame for his discovery. He was appointed Professor of Protobiology at Yale University Medical Center, and was also on the staff of the Pasteur Institute. In 1931 he gave a series of monthly lectures on phage therapy to the New YorkAcademy of Medicine. He established phage therapy centers in several countries, including the U. S., France, and Soviet Georgia. A fictionalized account of his work was depicted in rrowsmith, the Pulitzer-prize winning novel by Sinclair Lewis.
There are many attributes of phages (see Table 1) that would tend to favor a positive outcome in therapy. Despite these attributes of phages, there were so many problems with the way phage therapy was practiced in the historical era that, by the time antibiotics were introduced in mid-century, it was already in sharp decline in the West. The investigators who developed antibiotics did not make the kinds of mistakes exhibited by the early phage investigators.
Key problems with phage therapy, and how the problems can be overcome
Problem 1. Host range
The issue. Phages tend to have a relatively narrow host range, posing certain disadvantages. A disadvantage is that one should administer only those phage strains shown to be strongly lytic for the bacterial strain infecting the given patient. If the patient’s condition is too critical to take the time required for this matching, then one should use a grouping (a panel) of phages, where each of the phages therein has a broad-enough host range that most strains of the bacterial target are likely to be targeted. In his lectures to the New York Academy of Medicine in 1931, d’Hérelle cited the reports of other colleagues whose initial trials used phages “off the shelf” (without being shown to be virulent for the bacteria infecting the patient) and had negative outcomes, but who did match the phage to the bacteria in subsequent trials and obtained positive outcomes.
The solution. 1) Screen the bacteria infecting a given patient against a panel of phages, to ensure that one of the phage strains will be lytic (analogous to the “culture and sensitivity test” that physicians should perform; and 2) develop “multivalent” phages that lyse all or most of the bacterial strains within a given species of pathogen.
..........................................................................................................................
Table 1 - Attributes of phages that tend to favor a therapeutic response
Table 1 - Attributes of phages that tend to favor a therapeutic response
The Issue : Fate of the drug molecule
Limitations of Antibiotics : Metabolic destruction of the molecule, as it works
Advantages of phages: Exponential growth in numbers, so that the “ drug” makes more of itself at the site of infection, where it is needed
.....................
The Issue : Concentration of the "drug ” required to kill a given bacterium within the spectrum
Limitations of antibiotics: Numerous molecules of the antibiotic are needed to kill a given bacterium. During initiation of therapy (and between doses), the sub-lethal dose that bacteria “see'' affords them the opportunity to express resistance genes
Advantages of phages: “All or nothing” effect: one phage particle is sufficient to kill a given bacterium
...........................
The issue: Ability to overcome bacterial resistance
Limitations of antibiotics: Antibiotics are fixed, immutable chemicals that cannot adapt to a bacterial mutation and therefore become obsolete. Bacteria that have resisted them can pass along the resistance trait within and between species
Advantages of phages: Phages are “living” organisms that undergo mutations, some of which can overcome bacterial mutations. E. g., mutated phage tail fibers can allow binding to a mutant bacterial receptor, or mutated phage DNA can escape cleavage by mutant bacterial endonucleases
....................
The issue: Spread of bacterial resistance
Limitations of antibiotics: The antibiotics in use tend to be broad spectrum, thereby provoking resistance in several species and genera of bacteria (in addition to the one targeted}
Advantages of phages: Although there are some exceptions, phages tend not to cross species boundaries. Thus even though the targeted bacterial species may become resistant to the phage, it is unlikely that other species will.
Limitations of Antibiotics : Metabolic destruction of the molecule, as it works
Advantages of phages: Exponential growth in numbers, so that the “ drug” makes more of itself at the site of infection, where it is needed
.....................
The Issue : Concentration of the "drug ” required to kill a given bacterium within the spectrum
Limitations of antibiotics: Numerous molecules of the antibiotic are needed to kill a given bacterium. During initiation of therapy (and between doses), the sub-lethal dose that bacteria “see'' affords them the opportunity to express resistance genes
Advantages of phages: “All or nothing” effect: one phage particle is sufficient to kill a given bacterium
...........................
The issue: Ability to overcome bacterial resistance
Limitations of antibiotics: Antibiotics are fixed, immutable chemicals that cannot adapt to a bacterial mutation and therefore become obsolete. Bacteria that have resisted them can pass along the resistance trait within and between species
Advantages of phages: Phages are “living” organisms that undergo mutations, some of which can overcome bacterial mutations. E. g., mutated phage tail fibers can allow binding to a mutant bacterial receptor, or mutated phage DNA can escape cleavage by mutant bacterial endonucleases
....................
The issue: Spread of bacterial resistance
Limitations of antibiotics: The antibiotics in use tend to be broad spectrum, thereby provoking resistance in several species and genera of bacteria (in addition to the one targeted}
Advantages of phages: Although there are some exceptions, phages tend not to cross species boundaries. Thus even though the targeted bacterial species may become resistant to the phage, it is unlikely that other species will.
Numerous molecules of the antibiotic are needed to kill a given bacterium. During initiation of
therapy (and between doses), the sub-lethal dose that bacteria “see” affords them the opportunity to express resistance genes. Antibiotics are fixed, immutable chemicals that cannot adapt to a bacterial mutation and therefore become obsolete. Bacteria that have resisted them can pass along the resistance trait within and between species.
therapy (and between doses), the sub-lethal dose that bacteria “see” affords them the opportunity to express resistance genes. Antibiotics are fixed, immutable chemicals that cannot adapt to a bacterial mutation and therefore become obsolete. Bacteria that have resisted them can pass along the resistance trait within and between species.
The antibiotics in use tend to be broad spectrum, thereby provoking resistance in several species and genera of bacteria (in addition to the one targeted) . Exponential growth in numbers, so that the “drug” makes more of itself at the site of infection, where it is needed.
“All or nothing” effect: one phage particle is sufficient to kill a given bacterium. Phages are “living” organisms that undergo mutations, some of which can overcome bacterial mutations. E. g., mutated phage tail fibers can allow binding to a mutant bacterial receptor, or mutated phage DNA can escape cleavage by mutant bacterial endonucleases Although there are some exceptions, phages tend not to cross species boundaries. Thus even though the targeted bacterial species may
become resistant to the phage, it is unlikely that other species will."
....................................................................................................................
Problem 2. Bacterial debris present in the phage preparations
Problem 2. Bacterial debris present in the phage preparations
The issue. Injection of even minute amounts of endotoxin and other bacterial debris can be fatal to patients. Unfortunately, many of the phage preparations used by practitioners in the historical era were crude lysates. When these preparations were injected i.v., i.p., and in some cases even intrathecally, any beneficial effect of the phages would likely have been counteracted by illness and deaths resulting from the endotoxin.
The solution. Modern technology allows density centrifugation, banding, and other methods of purification.
Problem 3. Attempts to remove host bacteria from therapeutic preparations
The issue: In order to ensure that phage preparations would not contain live bacteria, some early investigators added mercurials and/or oxidizing agents, while others heated them. It is now known that such agents and procedures will denature or otherwise inactivate the phage coat proteins. These investigators did not check for continued viability of the phages. The false-negative results of such studies were the unintended (but inevitable) consequence of such practices.
The solution: Sterile filtration. If chemical agents must be used, reiterate the preparation over time to ensure that the phage remain viable.
Problem 4. Rapid clearance of phages
The issue. In fairness to phage investigators in the historical era, at the time it was not an accepted practice, in any discipline, to conduct pharmacokinetic studies. However, had the early phage investigators conducted such studies, they would have discovered that bacteriophages (being foreign proteins) tend to be rapidly cleared from the circulation. This clearance problem was first documented by Merril and his colleagues in 1973 who injected high titers of phage lambda into non-immune germ-free mice. They discovered that the phages were rapidly cleared by the spleen, liver and other filtering organs of the reticulo-endothelial system (RES)7.
This was a seminal observation, given Gunther Stent’s widely-accepted statement that one of the principal reasons phages had failed as a therapeutic was their supposed inactivation by pre-existing antibodies to them. However, any clearance of the phages from the bloodstream of the germ-free animals used by Merril and his group (ref.7) would not be due to antibodies, since those animals had never previously been exposed to bacteria or bacteriophages (and so would not have antibodies). Moreover, the phages in Merril’s experiment remained viable in the spleens of these animals over a period of several days, indicating that they were neither neutralized by antibody nor engulfed by macrophages. Rather, they appeared to have been passively entrapped in (sequestered by) these filtering organs. Such trapped phages would be unavailable to reach bacteria.
The solution. The author of this review collaborated with investigators at the U.S. National Institutes of Health (MERRIL et al.11) in the development of a method to isolate and amplify phage strains that are cleared at a slower rate. We reasoned that in all species of phage, minor variations in coat proteins might be present that would enable some variants to be less easily recognized by the RES organs and to thereby remain in the circulation for longer periods of time than the “average” wild-type phage. In this “serial passage” method, the wild-type preparation is injected into an animal, and then blood samples are taken at progressively longer time points. Any phages found in the blood sample are grown to high titer and reinjected. Through iterative rounds of passage, one can amplify the long-circulating strains being isolated. U.S. and PCT patents have been granted on this method.
For coliphage lambda as well as for salmonella phage P22, phage variants were isolated in this manner that were much longer-circulating than the wild-type. For example, for every 100 000 particles of the wild-type lambda used at baseline, only one particle remained in circulation at 18 h; whereas for the long-circulating phage mutant isolated at the 8th round of serial passage, for every 100 000 injected, at 18 h 62 500 particles remained in circulation. For each moment of time, far more of these long-circulating phages are propagating exponentially, as compared to the situation for the wild-type phages.
As predicted, these long-circulating phages were far superior to the wild-types from which they were derived, in terms of rescuing animals from an otherwise-fatal fulminant bacteremia: 1) with no treatment, all animals were dead within 48 h; 2) treatment with the wild-type phages prevented death, but the animals became critically ill (a human with such degrees of illness would be in the intensive care unit); and 3) in contrast, with administration of the long-circulating phage strain, the only sign of illness seen was mild lethargy. These results were published in the Proceedings of the National Academy of Sciences (ref. 11), and were accompanied by a Commentary by Nobel laureate Dr. JOSHUA LEDERBERG 8.
R. M. Carlton: Phage Therapy in the Past and Future P 269
We have elucidated the molecular basis of the mutation in lambda that reduced its rate of clearance: a single point mutation, an A to G transition, had occurred in the gene encoding the major head protein E.This mutation substituted a basic amino acid (lysine) for an acidic one (glutamic acid), causing a double charge shift readily seen on 2D gel electrophoresis.Computer modeling predicted that the mutation occurred in a loop of the E protein that sticks out into space and that therefore may interact with the external environment. A double charge shift in this region ofa protein that is highly represented on the surface of the virion could conceivably alter the phage’s interaction
with the microcirculation of the spleen, in such a waythat the mutant phage is less easily entrapped than thewild-type.
with the microcirculation of the spleen, in such a waythat the mutant phage is less easily entrapped than thewild-type.
Problem 5. Lysogeny
The issue. It was not until the late 1950s that Lwoff demonstrated the ability of some phage genomes to integrate into the bacterial chromosome as “prophages.”After a period of time (up to days or weeks, or longer), such prophages can enter the lytic cycle, and will thus appear as plaques on a bacterial lawn. It is likely that some phage therapy trials in the historic era had a negative outcome due to the inadvertent use of phage strains that, being lysogens, could not provide the rapid lysis and exponential growth in numbers that are needed for full efficacy.
The solution. Use only phages that are lytic; sequence phages that are strong candidates for clinical trials, looking for (among other things) homologies to known genes of lysogeny.
Problem 6. Anti-phage antibodies
The issue. There are reports in the literature20 that neutralizing antibodies appear a few weeks after administering phages to humans or animals. Given the time lag, antibodies would not seem likely to interfere with an acute treatment lasting a week or so. However, in chronic treatment, or in treatment of a recurrence of the same bacterial infection, the neutralizing antibodies might prevent some proportion of the administered dose of phages from being able to adhere to the bacterial target.
The solution. In treating chronic or recurrent infections it may be possible to administer a higher dose of phage, to compensate for those that are rendered non-viable by interaction with neutralizing antibodies. In any case, the types and titers of antibodies that develop should be systematically studied in humans.
Problem 7. Failure to establish scientific proof of efficacy
In scholarly reviews of comparative styles of research, Dutch historian TON VAN HELVOORT24 has discussed d’Hérelle’s systematic failure to conduct double-blind studies. As van Helvoort pointed out, while it is true that ethical problems are faced by anyone who has to administer placebo to some patients (in order to prove efficacy), nevertheless the investigators who later tested antibiotics did conduct double--blind, placebo-controlled trials. Van Helvoort points out that, even when using phages to treat an epidemic of diarrhea in poultry on a French farm, d’Hérelle failed to use a placebo on half the flock (a situation where ethical considerations would not have been an issue). As a consequence, all reports of phage therapy’s successes in the historical era were anecdotal. No systematic proof was available to demonstrate that the results were reliable and repeatable.
In scholarly reviews of comparative styles of research, Dutch historian TON VAN HELVOORT24 has discussed d’Hérelle’s systematic failure to conduct double-blind studies. As van Helvoort pointed out, while it is true that ethical problems are faced by anyone who has to administer placebo to some patients (in order to prove efficacy), nevertheless the investigators who later tested antibiotics did conduct double--blind, placebo-controlled trials. Van Helvoort points out that, even when using phages to treat an epidemic of diarrhea in poultry on a French farm, d’Hérelle failed to use a placebo on half the flock (a situation where ethical considerations would not have been an issue). As a consequence, all reports of phage therapy’s successes in the historical era were anecdotal. No systematic proof was available to demonstrate that the results were reliable and repeatable.
Problem 8. The scientific style of phage investigators in the historical era, D’Hérelle’s failure to conduct placebo-controlled studies, even on chickens, is an important example of his style. This story is a notable example of the negative impact an investigator’s personality can have on the outcome of a discovery, and d’Hérelle’s style contrasts sharply to the strongly positive influence that other scientists (such as Pasteur) have had on the outcomes of their discoveries. Whereas Pasteur excelled at conceiving of definitive experiments, and was persuasive in style, d’Hérelle failed to conduct definitive experiments, and was antagonistic rather than persuasive. For example, d’Hérelle maintained to the end that phages are the sole mechanism of defense against bacterial infection.
While he may have been correct in his view that epidemics can sometimes be checked by the spontaneous appearance of a lytic strain of phage, nevertheless he was incorrect in categorically dismissing the discoveries of Nobel laureates Metchnikoff and Ehrlich, who had shown that cellular elements (white blood cells) and humoral elements (antibodies and complement) constitute the innate host defenses against infection. D’Hérelle was afforded many opportunities to integrate his discovery with those of Metchnikoff and Ehrlich, but refused to the end (see below).
In addition to the damage he was doing to himself and his cause with this adamance, d’Hérelle was attacked by Nobel laureate Jules Bordet (for whom Bordetella pertussis was named), who had an intense dislike not just for d’Hérelle’s science but also for the man himself. Bordet used his considerable influence to discredit D’HÉRELLE 5.
D’Hérelle retreated from attacks by Bordet and others, and moved to Soviet Georgia in the 1930s (see ref. 13). An ardent communist, he dedicated the last of his published treatises to Josef Stalin. He was in Paris at the outbreak of World War II, refused to offer his skills with phage therapy to the Germans*, and spent the occupation years in prison. By the time of the liberation his health had been broken. He was invited to a post-War international scientific symposium, where colleagues made a last effort to see if they could help him bridge the gulf. He persisted in his belief that phages were the body’s sole mechanism of defense against bacteria (“Ce n’est que la phage…”), and he died in isolation in 1949. Surely the prospects of phage therapy in the historical era would have been better served if d’Hérelle had possessed some of the personality traits and scientific style of Pasteur.
* The push of the German army into the region of Georgia was intended not only to capture the region’s oil wells, but also to obtain the collection of phages manufactured at the Eliava-d’Hérelle Institute in Tblisi. That institute was providing phages to the Russian army, to control dysentery, Staphylococcus aureus infections ofwounds, and other bacterial problems associated with war
Animal Models of Phage Therapy
From the 1950s to the 1980s there was little published on the subject of phage therapy. Then papers began to appear demonstrating the utility of phage therapy in animal models. For example, phages were shown to be effective in rescuing rats from fatal systemic infections (induced with E. coli)14 in rescuing calves and lambs from fatal diarrhea (induced with E. coli)15, 16, in rescuing chicks from fatal diarrhea (induced with S. typhimurium) 4, and in preventing destruction of skin grafts in burned rabbits by Pseudomonas aeruginosa18. As mentioned above MERRIL et al.11 demonstrated in 1996 that mice with fulminant E. coli bacteremia could be rescued by phages, and that long-circulating phage variants were superior to the wild-types (see below). In one of those studies cited, Smith and Huggins (ref. 6) demonstrated that, in rats inoculated with a lethal intramuscular dose of E. coli, a single injection of a phage preparation was more effective than multiple injections of antibiotics (chloramphenicol, tetracycline, etc.). This work was replicated in 1997 by LEVIN and BULL9, who used mathematical modeling in a population dynamics approach to study the titers of phages and bacteria in the animals. The investigators concluded that the reason a single injection of phage was
superior to multiple injections of antibiotics was that the phages grew exponentially in number, overwhelming the bacteria present.
superior to multiple injections of antibiotics was that the phages grew exponentially in number, overwhelming the bacteria present.
Current Status of Human Phage Therapy Efforts
Poland. Phage therapy is practiced in Poland, albeit on a small scale. In the mid-1980s a series of papers was published by a group led by the late Prof. S. S´lopek and his colleagues, including Dr. M. Mulczyk and Dr. B. Weber-Da˛browska, working at the L. Hirszfeld Institute of Immunology and Experimental Therapy (a branch of the Polish Academy of Sciences). These papers20–23 reported on 550 cases of suppurative bacterial infections (empyemas, peritonitis, osteomyelitis, etc.) in humans. Most of the cases were chronic; most were resistant to all available antibiotics; and most had not been referred for this form of therapy until all else had failed, meaning that it was often quite late in thedisease progression. The bacterial pathogens targeted included Staphylococcus aureus, Pseudomonas aeruginosa, Klebsiella pneumoniae and E. coli. The phages used by these investigators are reported to have cured approximately 90% of the cases.
The criteria of cure were cessation of suppuration and,where applicable, complete closure of wounds/fistulae (many of which had been draining for months). These investigators administer phages orally, because they are aware of the hazards of administering them parenterally (not all of the bacterial debris has been removed). They pre-treat the patients with antacids and gelatin in order to protect the phages from destruction by gastric acidity. These same investigators have published evidence that phages administered orally to humans in this manner do in fact reach the bloodstream 26.
The Polish investigators have been rigorous in matching the phages to the bacterial strain infecting the given patients. Their practice, as stated in the published reports, is to culture the bacteria during the course of treatment, so that the occurrence of a mutant resisting the phage can be countered by switching to a different phage strain. The group also has panels of multivalent
phages available, for use in fulminant infections (such as septicemia with acute respiratory distress syndrome) where time is insufficient to classify the offending bacteria or to match phages to bacteria.
The group now has statistics on the treatment of approximately 1 300 cases. The overall cure rate across the spectrum of pathogens and sites of infection is approximately 86% (personal communication from Dr. B. Weber-Dabrowska). A criticism of the work by Slopek’s group is that - the absence of placebo controls means the power of suggestion cannot be definitively ruled-out. It is clear that the difficulties of that nation’s economy over recent decades has denied the investigators the financial resources needed to enroll matched cohorts in a placebo arm of a clinical trial. While the criticism is valid, and absolute proof of principle can be obtained only through placebo-controlled trials, nevertheless the usefulness of the data is improved by the detailed statistical accounting of the percentages of complete, partial and nil response. One of the factors that enables this author to find the data from Poland more believable (even in the absence of double-blind proof) is that in conditions such as emphysema where phage efficacy might be somewhat impeded, the group’s statistics show that the success rate is considerably lower than for other conditions where such impediments do not obtain*.
The Republic of Georgia.
The work started in Tblisi in the 1930s by d’Hérelle and his Georgian colleague, Eliava, continues to this day. In the 1970s, under the direction of Dr. Teimuraz Chanishvili, the Eliava-d’Hérelle Institute had a large staff manufacturing considerable quantities of phage preparations per year, primarily for the control of dysentery in the troops of the Soviet Army. This group has anecdotal evidence of the efficacy of phage therapy. They report, for example, that in certain adult and pediatric hospitals it is routine for their phage preparations to be administered topically on surgical incisions. Given the lack of statistical analysis, there is little to be said other than the anecdotal reports are encouraging that phage therapy can be useful.
Multidrug-Resistant (MDR) Bacteria Have Created a Need for Phage Therapy
Several species of bacteria have become resistant to most antibiotics, with some strains being resistant to all antibiotics. One example is vancomycin-resistant Enterococcus faecium (VRE), a low-virulence pathogen that now frequently causes fatal bacteremias due to complete resistance 2.Another example is vancomycin intermediate-resistant Staphylococcus aureus (VISA), strains of which have recently emerged in three nations (Japan, U.S. and Scotland), and are known to have killed 4 patients to date. Such strains spread throughout Japanese hospitals within a year of their first appearance.
Unfortunately, it has been demonstrated that some hospital strains of methicillin-resistant S. aureus (MRSA) that are widespread have become vancomycin resistant upon exposure of the patients to vancomycin 1, 2.
Experts predict that S. aureus will progress to become completely resistant to vancomycin (the antibiotic of last resort for most strains of this pathogen), and that when this occurs, millions of people will die each year from infections that had until recently been fairly easy to control. Based on such developments and impending developments with pathogens such as MRSA and VRE, opinion leaders have been warning that we are entering the “Post-Antibiotic Era”.
While pharmaceutical companies are developing new antibiotics to counter the trend, it has been shown that half a century of global antibiotic abuse has equipped the surviving bacteria with “supergenes” that enable them to quickly resist new classes of antibiotics, even those to which they have never been exposed 1.
Examples of the “supergenes” are mutations that 1) enable bacteria to pump out several classes of antibiotics (through an efficient efflux pump), or that 2) alter the antibiotic binding sites on ribosomal subunits, so that several different classes of antibiotics can no longer inhibit those subunits. As a consequence, in recent years, by the time newer antibiotics have gone through
clinical trials and have reached the market, 20% or more of clinical isolates in the hospitals are already resistant to them at the time of regulatory approval, and within a few more years the majority of strains are resistant.
Future Prospects for Phage Therapy
Infectious disease experts have warned that there is now a compelling need to develop totally new classes of antibacterial agents, ones that cannot be resisted by the same genes that render bacteria resistant to antibiotics.
Phage therapy represents such a “new” class. We believe that the impediments cited above (bacterial debris in the preparations, rapid clearance in the body, etc.) can be overcome, freeing up the phages so that their attributes (such as exponential growth, and the ability to mutate against resistant bacteria) can be used to great advantage.
There are 3 additional attributes of phages that should be noted: Host specificity. While the host specificity is somewhat of a drawback (requiring a match up of phage to bacterial target, and/or the development of highly multi-valent phages), it also offers the great advantage that the phages will not kill other species of bacteria.
* Conditions where phage efficacy is predicted to be reduced would include 1) hypoxic sites, where bacterial replication is slower and therefore phage replication is reduced; and 2) chronic obstructive pulmonary disease, where high acidity and proteases would be expected to inactivate some percentage of the phages.
Thus, e.g., phage therapy is not likely to kill off the healthy flora of the intestines, lungs or urogenital tract, and it is therefore unlikely to provoke the illnesses and deaths seen when antibiotics cause overgrowth of pathogens (such as Clostridia difficile and Candida albicans).
Genetic engineering. It is possible to genetically engineer phages to express new traits of potential value. In so doing, scientists will have to deal with the legitimate concerns of regulatory agencies concerning recombinant organisms. The regulatory obstacles may be well worth the price, given the powerful engineering tools that are currently available.
Ideal candidates for co-therapy with antibiotics. If a given bacterium acquires resistance to a phage (e.g. by a mutation in the receptor site or in the endonuclease enzymes), that mutation is not likely to “teach” the bacterium to resist the antibiotics (which do not target those structures). Similarly, if a given bacterium acquires resistance to an antibiotic (e. g. by a mutation in the reflux pump or in the ribosomal subunits), that mutation is not likely to “teach” the bacterium to resist the phage (which does not target those structures). Thus, if the bacterium is exposed to both agents, the odds are remote that any resistance genes it starts to express (or acquires anew) will enable it to survive. There are reports that bacteria tend to mutate against antibiotics once in every 106 divisions, while they tend to mutate against phages once in every 107 divisions.
Therefore the odds of a given bacterium mutating against a phage and an antibiotic at the same time would be the product of 106×107, meaning it would likely take 1013 bacterial divisions for such a double mutation to occur. Given that low probability, the co-administration of phages and antibiotics may help prevent the emergence of bacterial resistance to antibiotics, thereby greatly prolonging their clinical usefulness (and vice versa). Just as multiple classes of anti-HIV medications are administered to AIDS patients, to prevent the emergence of resistant strains of that virus, so it is that co-therapy with phages and antibiotics may also prove to be of great clinical value.
Conclusion
Multidrug-resistant bacteria have opened a second window for phage therapy. Modern innovations, combined with careful scientific methodology, can enhance mankind’s ability to make it work this time around. Phage therapy can then serve as a stand-alone therapy for infections that are fully resistant. It will also then be able to serve as a co-therapeutic agent for infections that are still susceptible to antibiotics, by helping to prevent the emergence of bacterial mutants against either agent.
References
1. ACKERMANN H. -W. and DUBOW M. (1987): Viruses of prokaryotes
I: General properties of bacteriophages (chapter 7). Practical
applications of bacteriophages. CRC Press, Boca Raton,
Florida.
2. ALISKY J. et al. (1998): Bacteriophages show promise as antimicrobial
agents. J. Infect., 36, 5–15.
3. BARROW P. A. and SOOTHILL J. S. (1997): Bacteriophage therapy
and prophylaxis: rediscovery and renewed assessment of
the potential. Trends Microbiol., 5, 268–271.
4. BERCHIERI A. et al. (1991): The activity in the chicken alimentary
tract of bacteriophages lytic for Salmonella typhimurium.
Res. Microbiol., 142, 541–549.
5. D’HÉRELLE F. (1917): Sur un microbe invisible antagoniste des
bac. dysentériques. Crit. Rev. Acad. Sci. Paris, 165, 373.
6. D’HÉRELLE F. (1922): The bacteriophage: its role in immunity.
Williams and Wilkens Co. /Waverly Press, Baltimore, USA.
7. GEIER M., FRIGG M. E. and MERRIL C. (1973): Fate of bacteriophage
lambda in non-immune germ-free mice. Nature, 246,
221–222.
8. LEDERBERG J. (1996): Commentary. Proc. Natl. Acad. Sci.
USA, 93, 3167–3168.
9. LEVIN B. and BULL J. J. (1996): Phage therapy revisited: the
population biology of a bacterial infection and its treatment
with bacteriophage and antibiotics. Am. Naturalist, 147, 881–898.
10. LEVY S. (1992): The antibiotic paradox. Plenum Press, New
York.
11. MERRIL C. et al. (1996): Long-circulating bacteriophage as
antibacterial agents. Proc. Natl. Acad. Sci. USA, 93, 3188–
2192.
12. MURRAY B. (1998): Diversity among multidrug-resistant Enterococci.
Emerging Infect. Dis., 4, 37–47.
13. SHRAYER D. (1996): Felix d’Hérelle in Russia. Bull. Inst. Pasteur,
94, 91–96.
14. SMITH H. W. and HUGGINS R. B. (1982): Successful treatment
of experimental E. coli infections in mice using phage: its general
superiority over antibiotics. J. Gen. Microbiol., 128, 307–318.
15. SMITH H. W. and HUGGINS R. B. (1983): Effectiveness of
phages in treating experimental E. coli diarrhoea in calves, piglets
and lambs. J. Gen. Microbiol., 129, 2659–2675.
16. SMITH H. W. and HUGGINS R. B. (1987): The control of experimental
E. coli diarrhea in calves by means of bacteriophage.
J. Gen. Microbiol., 133, 1111–1126.
17. SMITH T. et al. (1999): Emergence of vancomycin resistance in
Staphylococcus aureus. N. Engl. J. Med., 340, 493–501.
18. SOOTHILL J. S. (1992): Treatment of experimental infections of
mice with bacteriophages. Med. Microbiol., 37, 258–261.
19. SUMMERS W. C. (1998): D’Hérelle. Yale University Press (in
press).
20. S´LOPEK S. and KUCHAREWICZ-KRUKOWSKA A. (1987): Immunogenic
effect of bacteriophage in patients subjected to phage
therapy. Arch. Immunol. Ther. Exp., 35, 553–561.
21. S´LOPEK S., KUCHAREWICZ-KRUKOWSKA A., WEBER-DA˛BROWSKA
B. and DA˛BROWSKI M. (1985): Results of bacteriophage
treatment of suppurative bacterial infections. IV. Evaluation of
R. M. Carlton: Phage Therapy in the Past and Future 273
the results obtained in 370 cases. Arch. Immunol. Ther. Exp.,
33, 219–240.
22. S´LOPEK S., KUCHAREWICZ-KRUKOWSKA A., WEBER-DA˛BROWSKA
B. and DA˛BROWSKI M. (1985): Results of bacteriophage
treatment of suppurative bacterial infections VI. Analysis of
treatment of suppurative staphylococcal infections. Arch. Immunol.
Ther. Exp., 33, 261–273.
23. S´LOPEK S., WEBER-DA˛BROWSKA B., DA˛BROWSKI M. and KUCHAREWICZ-
KRUKOWSKA A. (1987): Results of bacteriophage
treatment of suppurative bacterial infections in the years 1981–
1986. Arch. Immunol. Ther. Exp., 35, 569–583.
24. VAN HELVOORT T. (1992): Bacteriological and physiological
research styles in the early controversy on the nature of the
bacteriophage phenomenon. Med. Hist., 36, 243–270.
25. WALDVOGEL F. (1999): New resistance in Staphylococcus
aureus. N. Engl. J. Med., 340, 556–557.
26. WEBER-DA˛BROWSKA B., DA˛BROWSKI M. and S´ LOPEK S.
(1987): Studies on bacteriophage penetration in patients subjected
to phage therapy. Arch. Immunol. Ther. Exp., 35, 563–568.
Received in May 1999
Accepted in June 1999
274 R. M. Carlton: Phage Therapy in the Past and Future
.................................................
Bdellovibrio, a Predatory Bacteria that has Future Potential in Fighting the Diseases That Kill Us.
Dr. Liz Sockett. Liz
December 2004
.................................................
Bdellovibrio, a Predatory Bacteria that has Future Potential in Fighting the Diseases That Kill Us.
Dr. Liz Sockett. Liz
Bdellovibrio are natural, tiny predatory bacteria that have other bacteria as their main food. Their strategy is to break into bacterial cells, close up the hole behind them suck their guts out! Having dissolved the inside of
Chris - What has the genetic information told you, and how can they be used in fighting infection?
Liz - We found that Bdellovibrio have lots of genes that make bacteria-dissolving enzymes. They also secrete a juice that breaks down chromosomes. These will both kill bacteria. Bdellovibrio don't look for any specific target sites on their bacteria prey, so there is no way for the bacteria to hide. Unfortunately, Bdellovibrio can't get into MRSA, but they can get into many others. We hope to use them on things like burns and leg ulcers, although more testing needs to be carried out. We might even be able to take genes and put them in the bacteria so they can attack MRSA.
Chris - What happens when the infection has cleared up?
Liz - If the Bdellovibrio burst out of the dead bacterium and can't find any more food, they just die. This makes it a self-terminating therapy that leaves no residue. They are likely to be best for wound infections, as eating them will kill good and bad stomach bacteria, and they also end up going down the loo [with unforeseen consequences].
.............................................
A useful predatory bacterium called Bdellovibrio bacteriovorus eats other bacteria (including important pathogens of humans, animals and crops). It attacks them from inside out using enzymes (called DD-endopeptidases) that first loosen the cell walls of prey bacteria and then cause them to round up like a pufferfish, providing space as a temporary home for the predator. However, Bdellovibrio also have similar cell walls so why don’t they fall victim of their own attack?
The project, funded by the Biotechnology and Biological Sciences Research Council (BBSRC), found that the bacterium uses an ankyrin-type protein called Bd3460 as a shield. It binds to the tip of the enzyme weapons, nullifying their action until they are safely secreted out of the Bdellovibrio and into the prey bacteria.
Dr. Andrew Lovering and Ian Cadby at the University of Birmingham determined the structure of the ankyrin protein using X-ray crystallography and found that that it attaches to two DD-endopeptidase weapons to temporarily deactivate them.
“When I first showed this to Liz, she hit the nail on the head by describing it as a decorative “quiff” on top of the endopeptidase” said Dr Lovering. “This covers up the active site of the enzymes that are used to cut cell walls and offers protection to the Bdellovibrio until these weapons are excreted into the prey.”
Carey Lambert, Rob Till and Prof Liz Sockett at the University of Nottingham confirmed the antidote protein’s use when the gene responsible for its production was deleted.
Prof Liz Sockett: “When the bd3460 gene responsible for antidote production was deleted, the Bdellovibrio had no way of protecting itself from its own weapons. When it attacked harmful bacteria with its cell-wall-damaging enzymes it also felt the effects.
“The Bdellovibrio bacteria lacking the bd3460 gene tried to invade the bacteria but suddenly rounded up like pufferfish and couldn’t complete the invasion – the fatter predator cell could not enter the prey cell.”
This is the first paper to discover a ‘self-protection’ protein in predatory bacteria.
Prof Liz Sockett added, “Most bacteria are not predatory and so understanding these mechanisms gives us a glimpse of how predation evolved. In this case it seems that the bd3460 gene was transferred into ancestors of Bdellovibrio, probably when they were beginning to develop as predators.”
Commenting on the potential impact of the study, Dr Andrew Lovering added: “If we are to use Bdellovibrio as a therapeutic in the future, we need to understand the mechanisms underpinning prey killing and be sure that any self-protective genes couldn’t be acquired by pathogens, causing resistance. Brilliantly, Liz and Carey have demonstrated this did not happen with the bd3460 antidote protein, and Ian and I showed how the mechanism works on predator enzymes only – this is a great inter-university collaboration.”
BBSRC is one of the UK Research Councils. The Research Councils, led by the Medical research Council, are working on a joint Antimicrobial Resistance (AMR) Programme to find new ways to kill infectious pathogenic bacteria that are drug-resistant. Bdellovibrio is a natural predator and understanding the mechanisms it requires for successful predation provides vital knowledge towards these aims.
.........................................................
https://www.nature.com/articles/nrmicro959
Opinion
Bdellovibrio as therapeutic agents: a predatory renaissance?
- Nature Reviews Microbiology volume 2, pages 669–675 (2004)
- doi:10.1038/nrmicro959
- Download Citation
- Published:
In the fight against antimicrobial resistance,
scientists discover how bacterial predators evolved to kill other
bacteria without harming themselves.
- Bacteria-killing bacteria (“predatory bacteria”) may assist humans in fighting pathogens in the post-antibiotic era
- How predatory bacteria function has been little understood to date
- Predators have been found to produce a protein “antidote” that protects them from their own weapons
- Self-protection technique allows one bacterium to destroy others
- The findings offer clues to how bacterial predation may have first evolved
- Understanding how these predators attack bacteria could provide new ways of combatting antimicrobial resistance
A useful predatory bacterium called Bdellovibrio bacteriovorus eats other bacteria (including important pathogens of humans, animals and crops). It attacks them from inside out using enzymes (called DD-endopeptidases) that first loosen the cell walls of prey bacteria and then cause them to round up like a pufferfish, providing space as a temporary home for the predator. However, Bdellovibrio also have similar cell walls so why don’t they fall victim of their own attack?
The project, funded by the Biotechnology and Biological Sciences Research Council (BBSRC), found that the bacterium uses an ankyrin-type protein called Bd3460 as a shield. It binds to the tip of the enzyme weapons, nullifying their action until they are safely secreted out of the Bdellovibrio and into the prey bacteria.
Dr. Andrew Lovering and Ian Cadby at the University of Birmingham determined the structure of the ankyrin protein using X-ray crystallography and found that that it attaches to two DD-endopeptidase weapons to temporarily deactivate them.
“When I first showed this to Liz, she hit the nail on the head by describing it as a decorative “quiff” on top of the endopeptidase” said Dr Lovering. “This covers up the active site of the enzymes that are used to cut cell walls and offers protection to the Bdellovibrio until these weapons are excreted into the prey.”
Carey Lambert, Rob Till and Prof Liz Sockett at the University of Nottingham confirmed the antidote protein’s use when the gene responsible for its production was deleted.
Prof Liz Sockett: “When the bd3460 gene responsible for antidote production was deleted, the Bdellovibrio had no way of protecting itself from its own weapons. When it attacked harmful bacteria with its cell-wall-damaging enzymes it also felt the effects.
“The Bdellovibrio bacteria lacking the bd3460 gene tried to invade the bacteria but suddenly rounded up like pufferfish and couldn’t complete the invasion – the fatter predator cell could not enter the prey cell.”
This is the first paper to discover a ‘self-protection’ protein in predatory bacteria.
Prof Liz Sockett added, “Most bacteria are not predatory and so understanding these mechanisms gives us a glimpse of how predation evolved. In this case it seems that the bd3460 gene was transferred into ancestors of Bdellovibrio, probably when they were beginning to develop as predators.”
Commenting on the potential impact of the study, Dr Andrew Lovering added: “If we are to use Bdellovibrio as a therapeutic in the future, we need to understand the mechanisms underpinning prey killing and be sure that any self-protective genes couldn’t be acquired by pathogens, causing resistance. Brilliantly, Liz and Carey have demonstrated this did not happen with the bd3460 antidote protein, and Ian and I showed how the mechanism works on predator enzymes only – this is a great inter-university collaboration.”
Notes to editor
Contact
Chris Melvin, 01793 414 694, Chris.melvin@bbsrc.ac.ukBBSRC is one of the UK Research Councils. The Research Councils, led by the Medical research Council, are working on a joint Antimicrobial Resistance (AMR) Programme to find new ways to kill infectious pathogenic bacteria that are drug-resistant. Bdellovibrio is a natural predator and understanding the mechanisms it requires for successful predation provides vital knowledge towards these aims.
.........................................................
..........................................................................................